Low water activity in food increases microbial heat resistance during heat sterilisation, making careful heating of these foods crucial. How much does microbial heat resistance increase as water activity decreases? This article summarises the impact of water activity on microbial heat resistance in foods.
For those unfamiliar with water activity before starting this article, please see the following article:
Water Activity Explained: What is It and How Does it Differ from Water Content?
The Relationship between Microbes, Heat Resistance, and Water Activity
Under low water activity conditions, microbial cells exhibit increased heat resistance. Numerous research reports have been published on this subject, especially concerning Salmonella, which poses significant survival challenges at low water activities.
The graph below illustrates the relationship between the heat resistance of Salmonella Enteritidis PT 30 and water activity. The experiment involved heating at 80°C while controlling relative humidity between 18–72% using silica dioxide granules as carriers in a specially designed test cell. The graph reveals the following: Salmonella shows increased D-values at 80°C as water activity decreases.
For those interested in the basics of microbial heating and D-values, please see the following article:
Food Heat Sterilization: Understanding and Applying Pasteurization, D Values, and Z Values
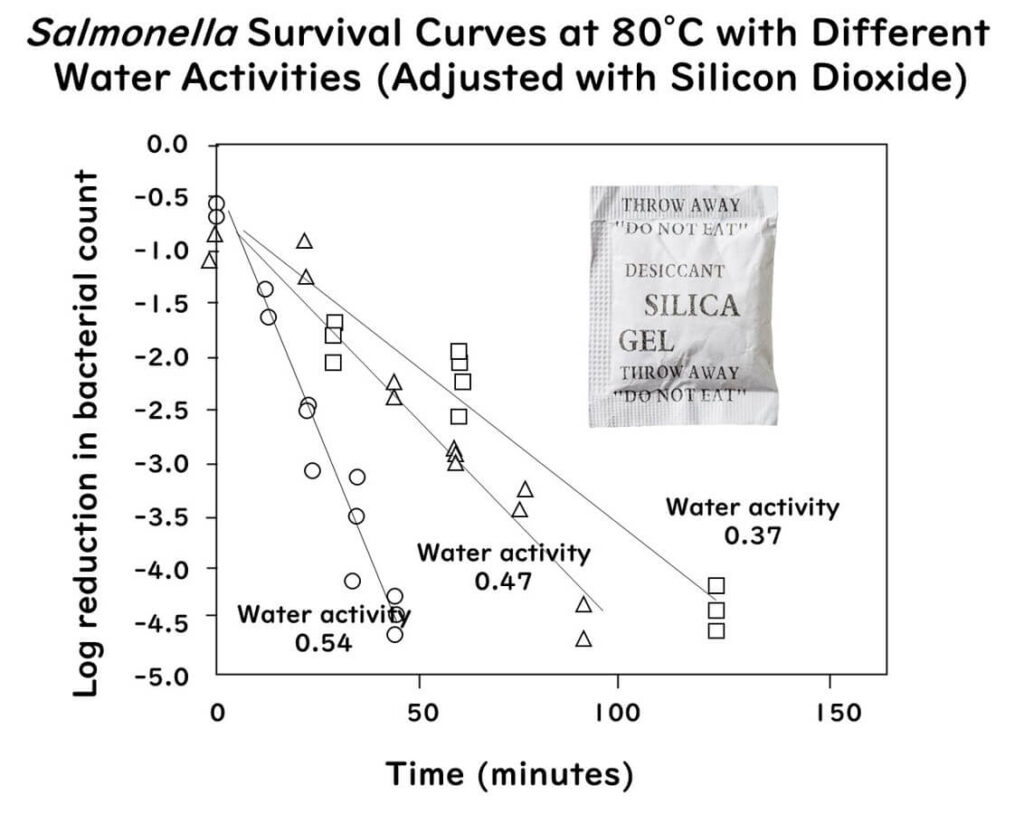
The graph above has been redrawn from the data partially extracted from the following paper:
Exponentially Increased Thermal Resistance of Salmonella spp. and Enterococcus faecium at Reduced Water Activity
Appl Environ Microbiol. 84(8):e02742-17(2018)
Increased Heat Resistance of Microbes in Various Low Water Activity Foods
How does changing water activity affect the heat resistance of Salmonella in actual foods? This section presents examples involving peanut butter, cinnamon powder, and almond powder.
Peanut Butter
Peanut butter, with a water activity below 0.35, does not support bacterial growth, thus it is unlikely to cause food poisoning. However, significant Salmonella outbreaks occurred in the United States from 2006 to 2012, prompting then-President Obama to criticise the FDA on an NBC show.
For more details on peanut butter-related Salmonella poisoning, see the following article:
Cavallaro E et al.,
Salmonella typhimurium infections associated with peanut products
N Engl J Med.2011 Aug 18;365(7):601-10. doi: 10.1056/NEJMoa1011208.
The graph below shows the decline of Salmonella Typhimurium in peanut butter (49% fat, 24% carbohydrates) adjusted to water activities of 0.2 and 0.4, heated at 90°C. It indicates that Salmonella's heat resistance increases at a lower water activity of 0.2.
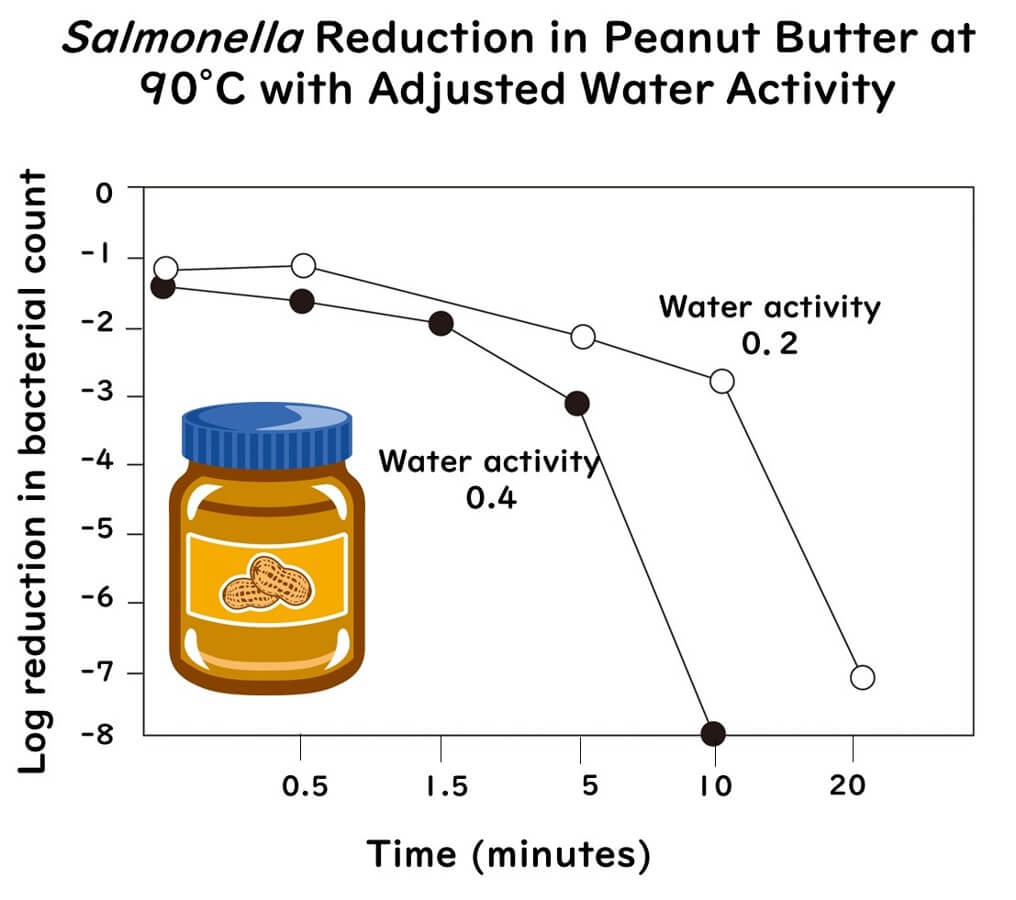
The graph above is plotted from data extracted from the following paper:
Increased Water Activity Reduces the Thermal Resistance of Salmonella enterica in Peanut Butter
Appl Environ Microbiol,79(15):4763-7( 2013 )
Spices (Cinnamon)
Reports of Salmonella contamination in spices like cinnamon are common. Between 2009 and 2010, 272 people in 44 US states fell ill from Salmonella poisoning linked to contaminated black and red pepper in salami.
For more details on this outbreak, see the following article:
Nationwide outbreak of Salmonella Montevideo infections associated with contaminated imported black and red pepper: warehouse membership cards provide critical clues to identify the source
Epidemiol. Infect. (2013), 141, 1244–1252.
The graph below shows the survival of Salmonella Enteritidis PT 30 in cinnamon powder, adjusted to different water activities and heated at 75°C. The log10 D-value of S. Enteritidis PT 30 decreases linearly as water activity increases.
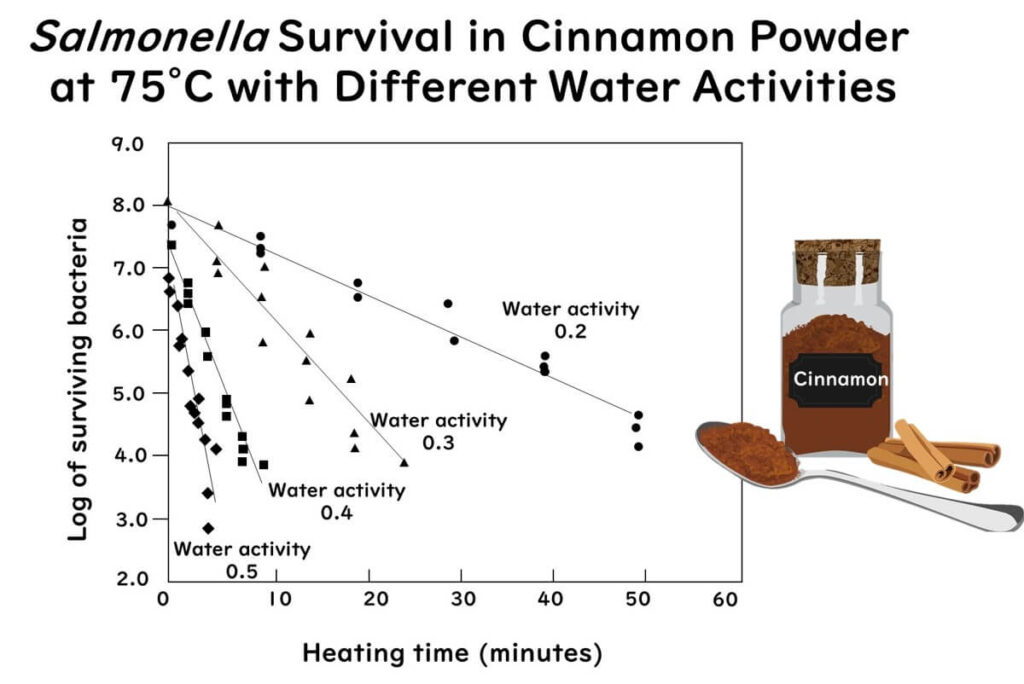
The graph above is plotted from data extracted and redrawn from the following paper:
Thermal inactivation of Salmonella Enteritidis PT30 in ground cinnamon as influenced by water activity and temperature
Food control v.124 pp. 107935(2021)
Almonds
The graph below shows the significant increase in heat resistance of Salmonella Enteritidis in almond kernels powder adjusted to water activities of 0.720 compared to 0.888.
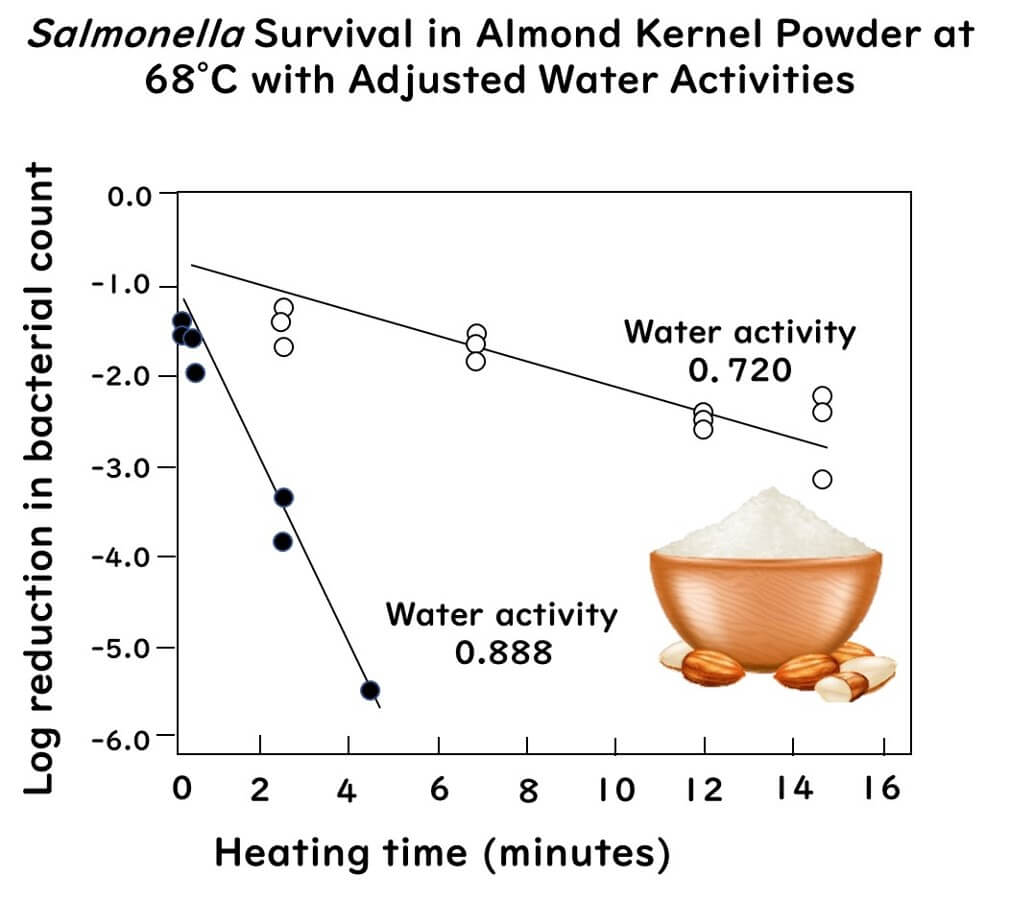
The graph above is drawn from data extracted from the following paper:
Thermal Inactivation of Salmonella Enteritidis PT 30 in Almond Kernels as Influenced by Water Activity
Journal of Food Protection 76(1):26-32(2013)
This research highlights the challenges of gently pasteurising raw almonds, which have been associated with multiple outbreaks of Salmonella poisoning in the US and Europe(CDC 2004).
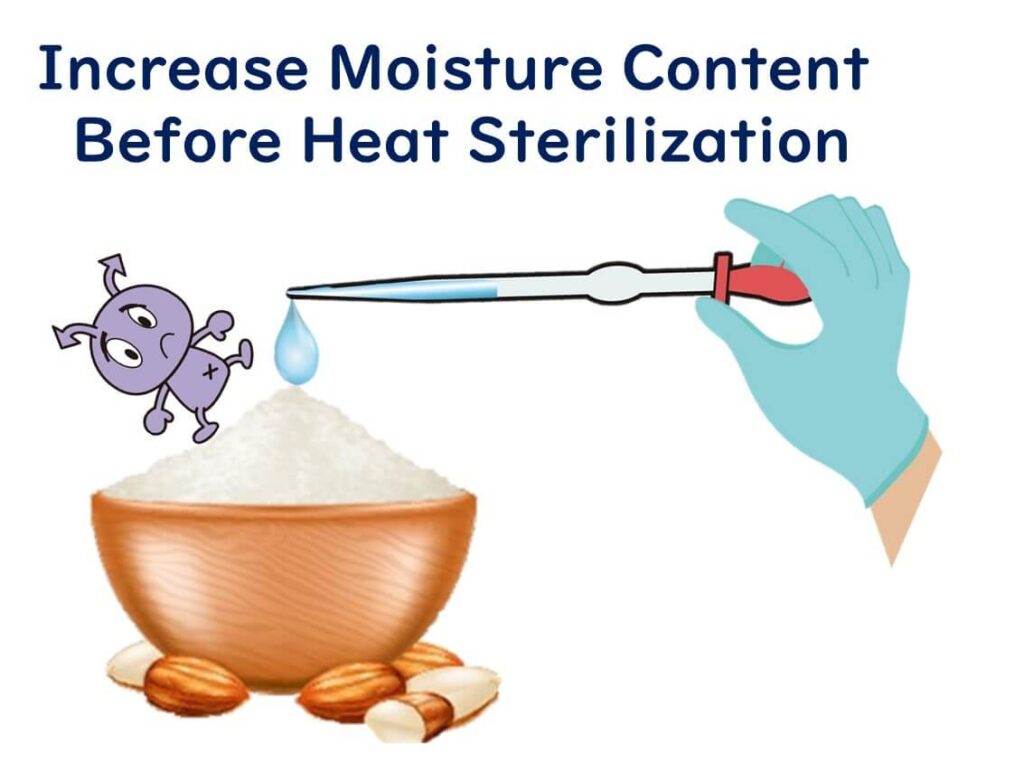
The results suggest that increasing the water content of almonds before a short, low-temperature treatment could efficiently sterilise the nuts—a method potentially applicable to other dried foods.
Microbial Heat Resistance May Be Determined Solely by Water Activity at the Time of Heating, Not Differences in Food Ingredients
Data from various researchers have shown that even with the same level of water activity, differences in food types can result in different heat resistance (D-values) for Salmonella. This has led to speculation that food components could influence Salmonella’s resistance under low water activity conditions. However, recent studies suggest that these differences are determined by changes in water activity during heating.
This section introduces a paper that detailed the effects of water activity on the heat resistance of Salmonella Enteritidis PT30 in three low water activity foods: flour, almond powder, and whey protein.
The experimental methods summarised are as follows:
- The heat resistance of Salmonella was evaluated in three types of food: flour, almond powder, and whey protein, each representing food groups rich in carbohydrates, fats, and proteins, respectively.
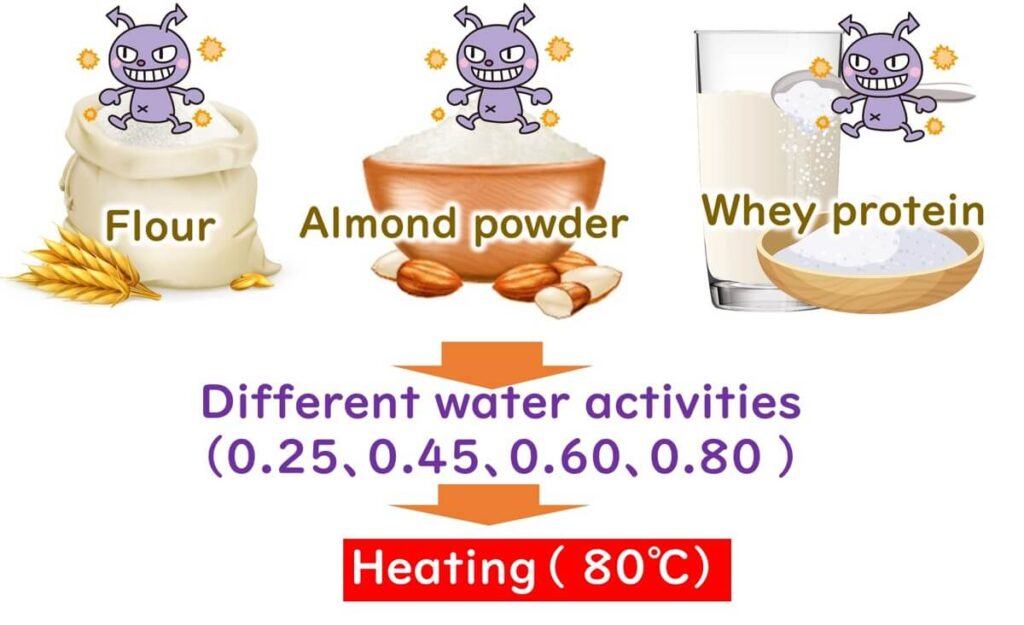
- The water activity of each powdered food was adjusted to 0.25, 0.45, 0.60, and 0.80 at room temperature (~20°C) using solutions of LiCl at different concentrations.
- The strain of Salmonella Enteritidis PT30, known for its relatively high heat resistance and associated with international outbreaks linked to raw almonds, was used for inoculation experiments.
- The samples were heated in aluminium cells at 80°C, and the D-values (the time required to inactivate 90% of the target bacteria) were measured.
The results were as follows:
- Salmonella was rapidly inactivated in all powders at higher water activities.
- The inactivation curves of Salmonella were grouped almost identically across the different types of food at the same water activity levels.
- Regardless of the food matrix or test method, the D-values for Salmonella at 80°C decreased exponentially with increasing water activity at the treatment temperature.
- The D-values at 80°C were almost identical among different powders at the same water activity. For example, the D-values for almond powder and whey protein at an aw of 0.50 at 80°C were 24.9 minutes and 23.2 minutes, respectively
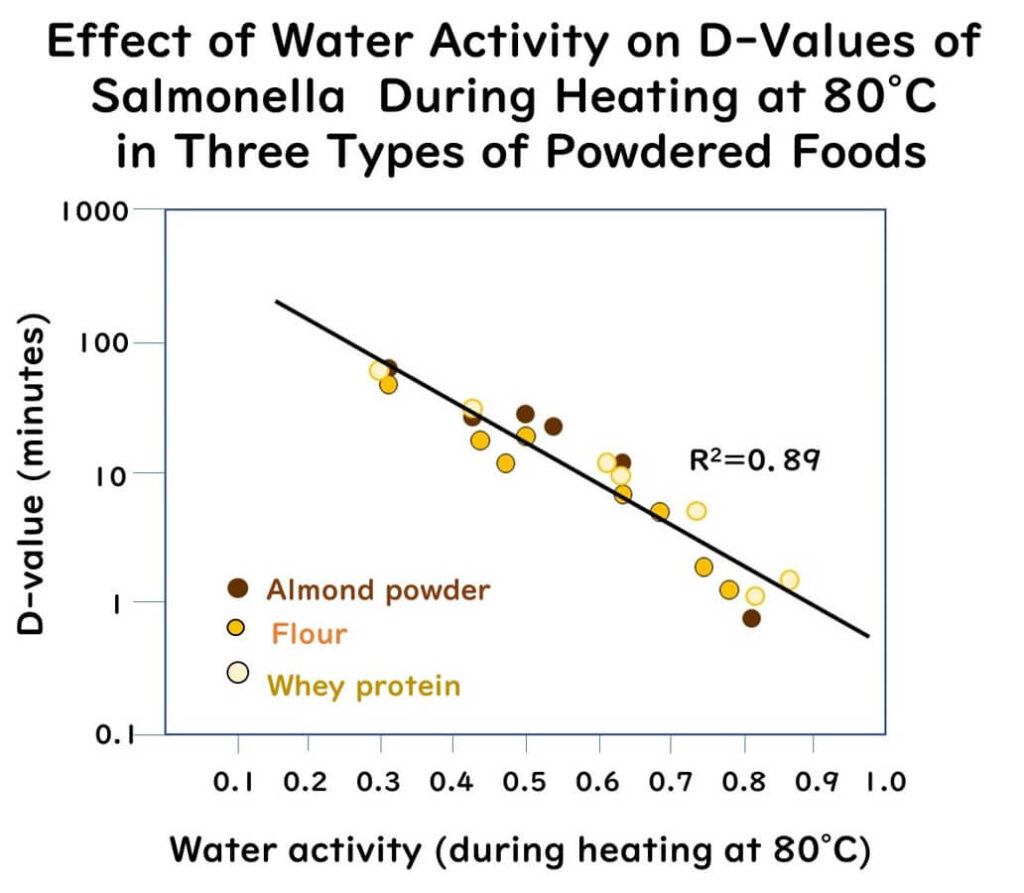
The graph above is redrawn from data extracted from the following paper:
High temperature water activity as a key factor influencing survival of Salmonella Enteritidis PT30 in thermal processing
Food Control, 98, 520-528(2019)
From these experimental results, the following conclusions were drawn:
- The differences in Salmonella’s D-values due to different foods are caused not by the food components themselves but by changes in water activity during heating brought about by those food components.
- Thus, the heat resistance of Salmonella is determined solely by water activity.
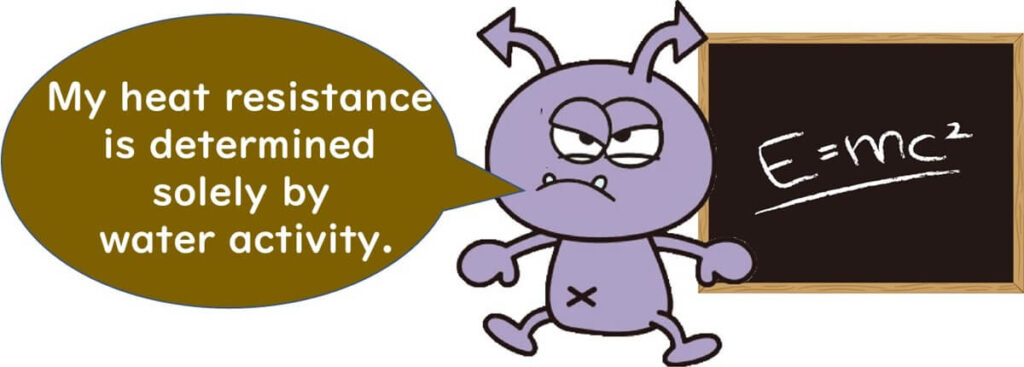
Mechanisms of Increased Heat Resistance in Microorganisms at Low Water Activity
Research has explored why low water activity imparts increased heat resistance to microbial cells. Below, some of the key hypothetical mechanisms proposed are outlined.
Heat Conductivity
Water is an excellent conductor of heat. Another article explains the significant differences in sterilisation efficiency between moist heat and dry heat sterilisation, so please see:
Sterilisation of Food: Grasping the Basics of Heat Sterilisation
For low-moisture foods, several studies have experimentally demonstrated that thermal conductivity decreases as moisture content decreases. A study by Tavman et al. investigated the thermal conductivity of 11 types of cheese, four types of yoghurt, and one type of butter. The research revealed that:
- Thermal conductivity increases linearly with increased moisture content, showing a very good correlation.
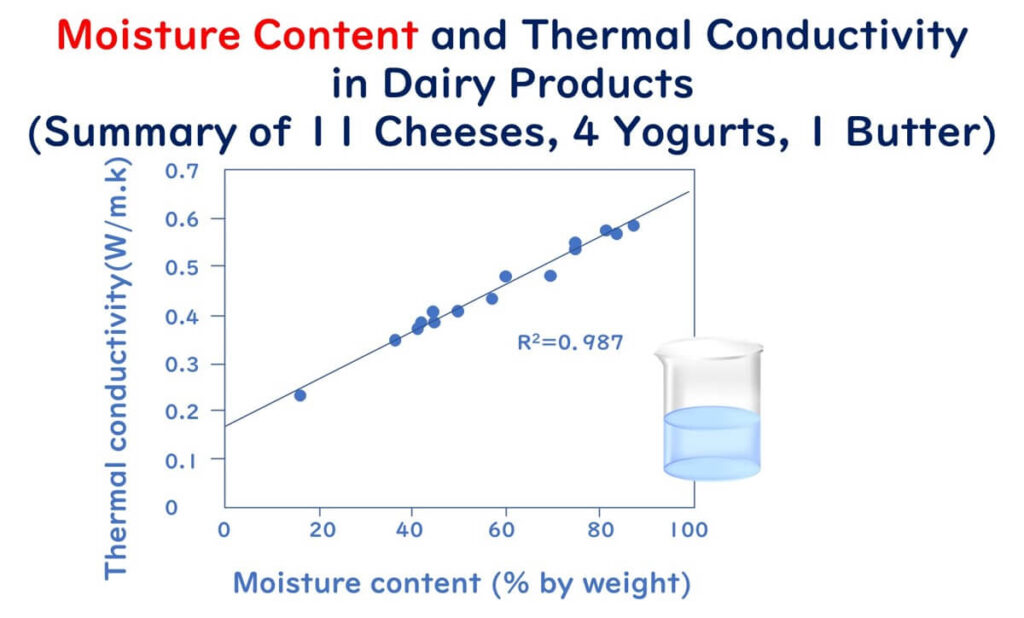
- Thermal conductivity decreases linearly with increased protein content in the food.
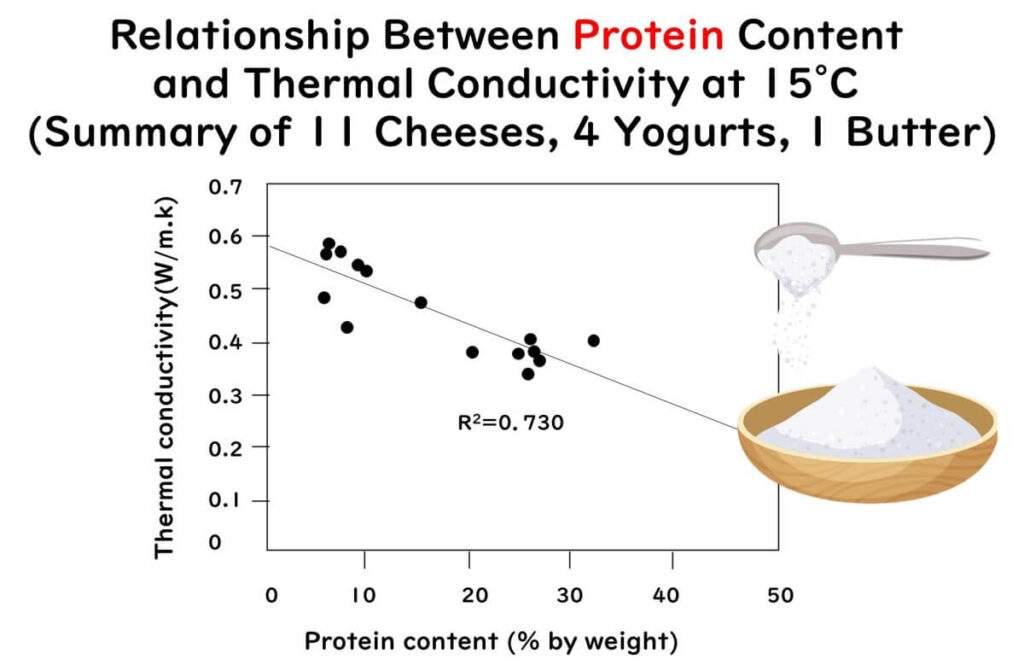
- Thermal conductivity decreases linearly with increased fat content in the food.
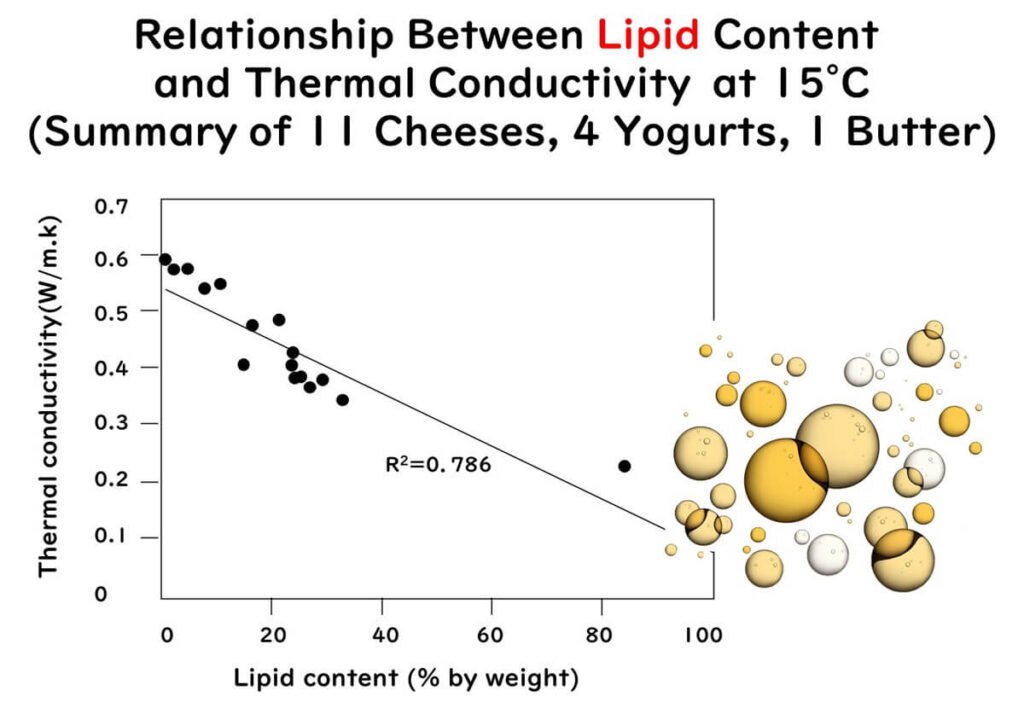
The three graphs mentioned above have been redrawn using partial data extracted from the figures and tables of the following paper:
Measurement of thermal conductivity of dairy products
Journal of Food Engineering 41 109-114(1999)
However, it is important to note that this paper primarily examines the relationship between moisture content and thermal conductivity, not water activity. Therefore, applying these findings directly to low water activity foods is not always 100% accurate. Yet, for many low-moisture content foods that are problematic in heat sterilisation, considering these conductivity differences often makes sense—except in high-moisture, low-activity foods like jams.
Protein Structure Rigidification Preventing Denaturation
When microbial cells are heated, water molecules begin to vibrate, weakening and breaking disulfide bonds and hydrogen bonds in surrounding proteins, causing changes in protein structures and denaturation. The main cause of bacterial cell inactivation under high moisture conditions is the irreversible destabilisation of ribosomes, particularly the 30S and 50S ribosome subunits, as experimentally verified by Lee and Kaletunc (2002).
Dynamic fluctuations in the three-dimensional structure of proteins are necessary for heat denaturation, which requires a sufficient amount of water.
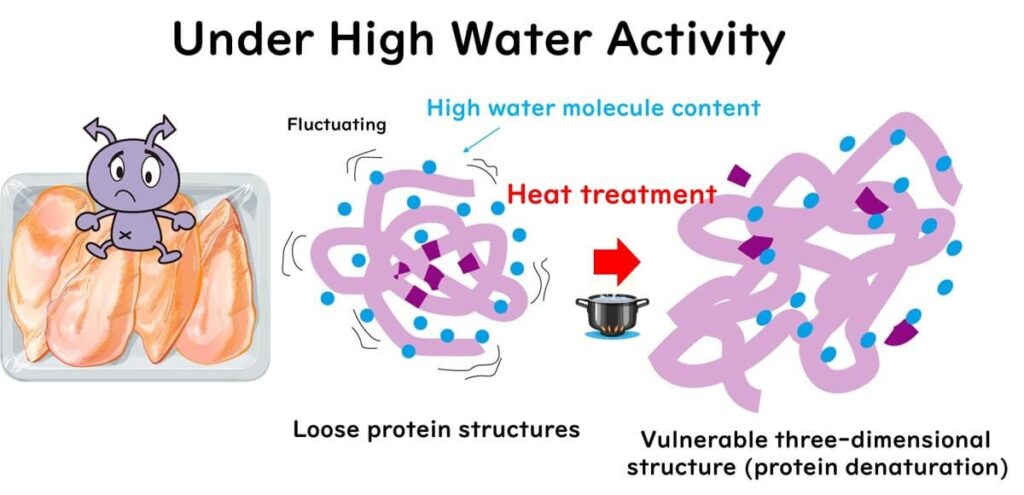
In low water activity conditions, these hydration waters decrease, resulting in the loss of structural flexibility in proteins. The loss of protein flexibility means that hydrophobic sites in enzymes become structurally rigid and buried, making them less likely to be exposed during heating. Therefore, low water activity makes it difficult to inactivate enzymes.
The high thermal resistance of Bacillus species and other heat-resistant spores is thought to be due to the low flexibility of their protein structures(Sunde et al、2009).
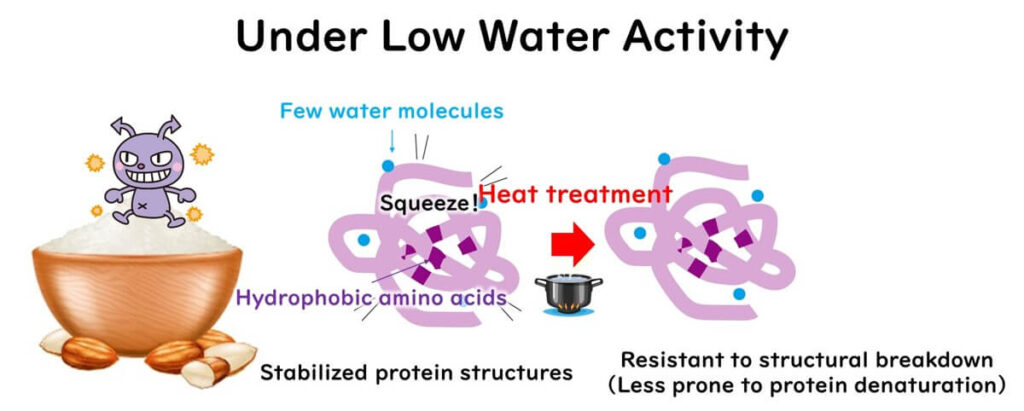
Various mechanisms for the increased heat resistance of microorganisms in low-water environments have been studied, but here only the representative hypotheses are noted.
Conclusion: Caution Needed in Sterilising Dry Foods
As discussed in this article, microorganisms exhibit significantly increased heat resistance in low-water-activity environments during heat sterilisation. Therefore, when heating dry or low-water-activity foods, care must be taken as the D-values obtained from liquid medium heating experiments may not be directly applicable. This necessitates heightened attention to ensure effective sterilisation without underestimating the resilience of these microbes.
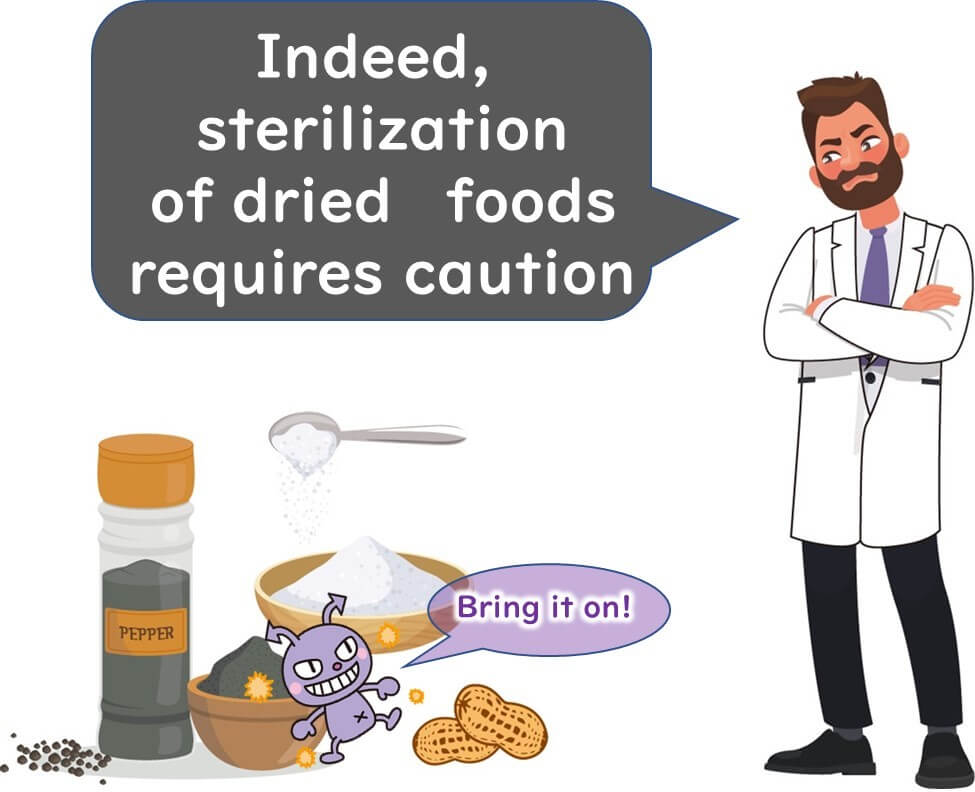