Ensuring cleanliness in food factories is crucial for product safety. The ATP test offers a fast and effective method to verify cleanliness after cleaning and sterilization, addressing the limitations of traditional microbial testing. This article explores the biological role and chemical structure of ATP, its efficiency as an energy carrier, and the practical methods and tools for ATP testing. By applying the ATP test effectively, food factories can achieve more efficient and comprehensive cleanliness management.
Introduction
After cleaning and sterilization, measuring the effectiveness of these processes is essential for maintaining proper cleanliness management. In food microbiology, microbial testing is often regarded as the most direct way to prevent secondary contamination. However, traditional microbial testing has limitations, including labor-intensive procedures and time delays, which make it unsuitable for routine and rapid checks.
In contrast, the ATP test provides a quick and straightforward method for evaluating cleanliness. By measuring the ATP levels on surfaces of equipment and the environment, this test offers a rapid and accessible solution for cleanliness assessment.
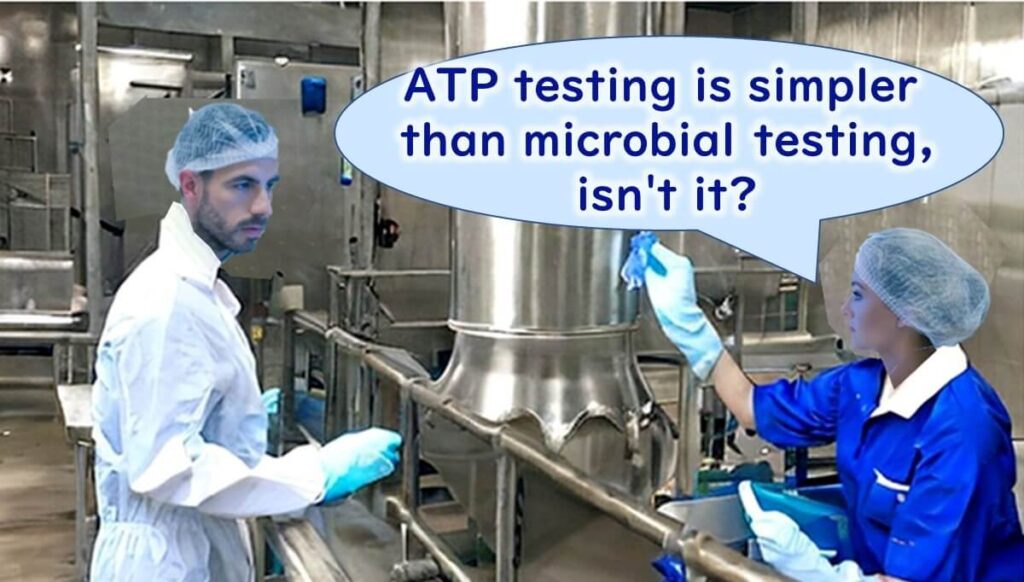
This article introduces the ATP test, highlights its differences from microbial testing, and explains its practical use in managing cleanliness in food factories.
The Biological Significance of ATP
Let’s begin with the basics.
Photosynthesis is a process where plants capture solar energy to create organic compounds. During this process, plants combine carbon dioxide and water to form sugars, which act as stored chemical energy—much like glue holding building blocks together.
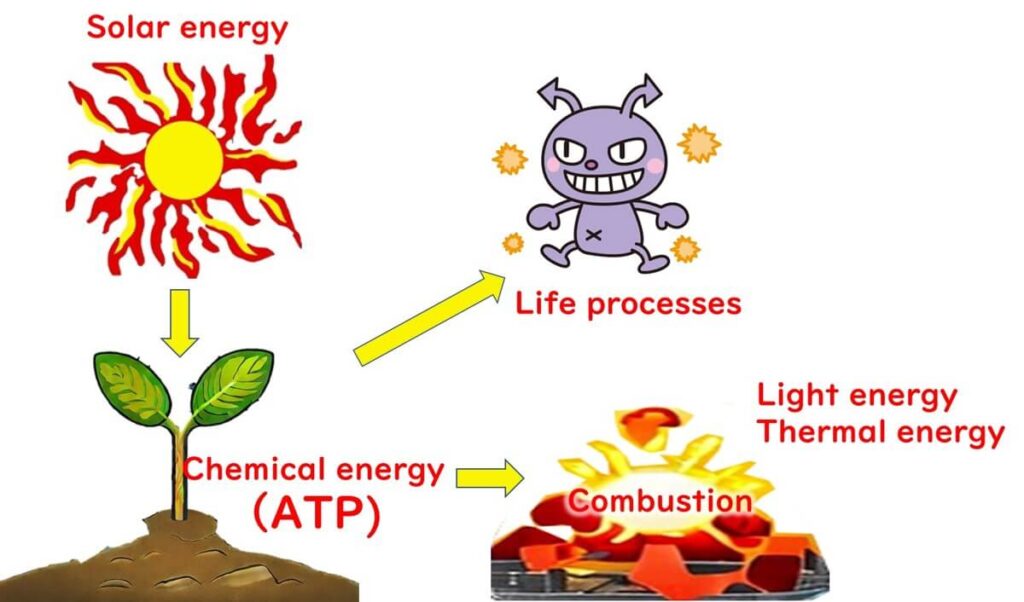
When plants metabolize, these sugars react with oxygen, releasing chemical energy as heat and light.
For heterotrophic organisms like animals and microbes, ATP serves as a universal energy currency. ATP is generated during the breakdown of sugars and other organic matter. It functions as a source of chemical energy, powering essential biological processes like muscle contraction and biomolecule synthesis.
In essence, plants release energy when they "burn" their stored sugars, while animals and microbes convert the chemical energy from plants into ATP, the primary energy currency that sustains life processes.
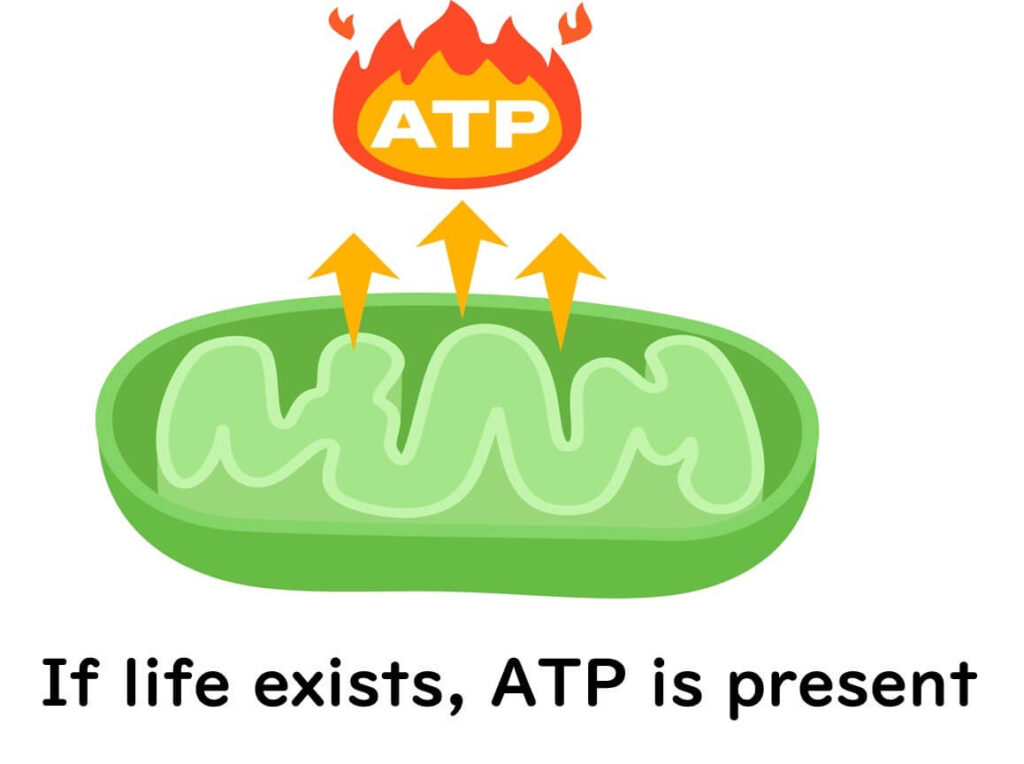
The Chemical Structure of ATP
ATP, or adenosine triphosphate, is composed of two key components: adenosine and three phosphate molecules. Adenosine itself is a nucleic acid base consisting of adenine and ribose. The three phosphate groups are linked to the ribose portion of adenosine. This structure forms the basis of ATP's remarkable role in energy transfer within living organisms.
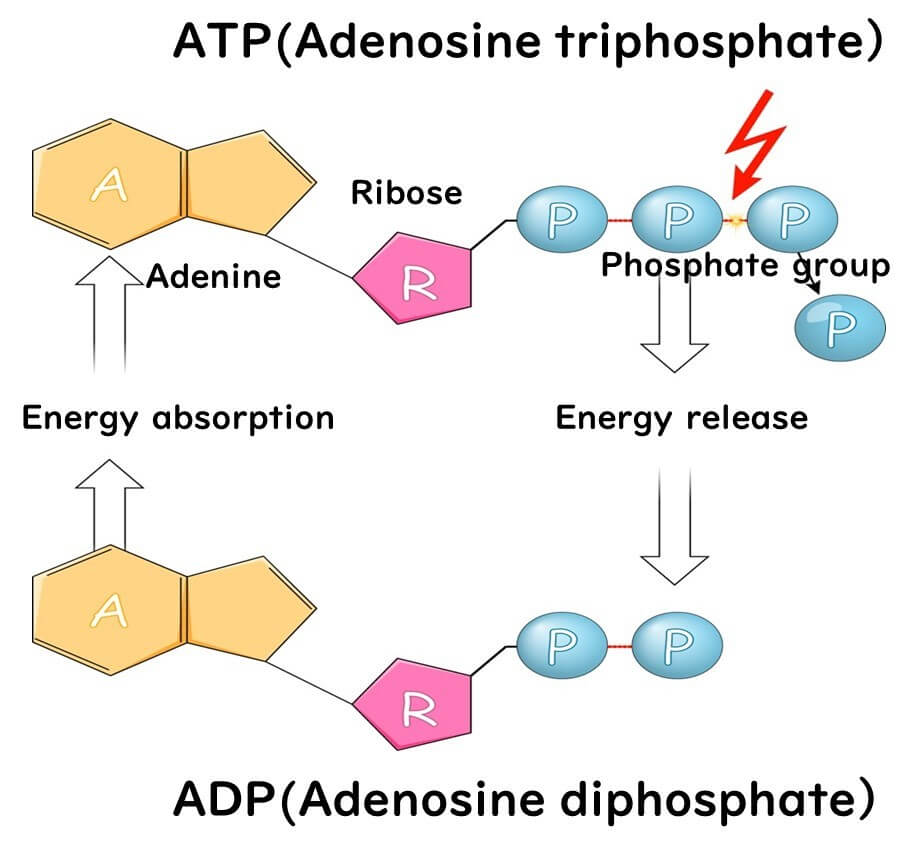
Why is ATP an Excellent Energy Carrier?
What makes ATP stand out as an energy carrier among countless compounds in the body?
The secret lies in the high-energy phosphate bonds within its structure. These bonds are formed between the three phosphate molecules, which are negatively charged and naturally repel each other. A significant amount of energy is required to hold these phosphates together, creating a state of high energy within the molecule.
When these phosphate bonds are broken, the stored energy is released in a burst, making ATP an efficient energy source. Despite being a low molecular weight compound, ATP can store substantial energy relative to its size.
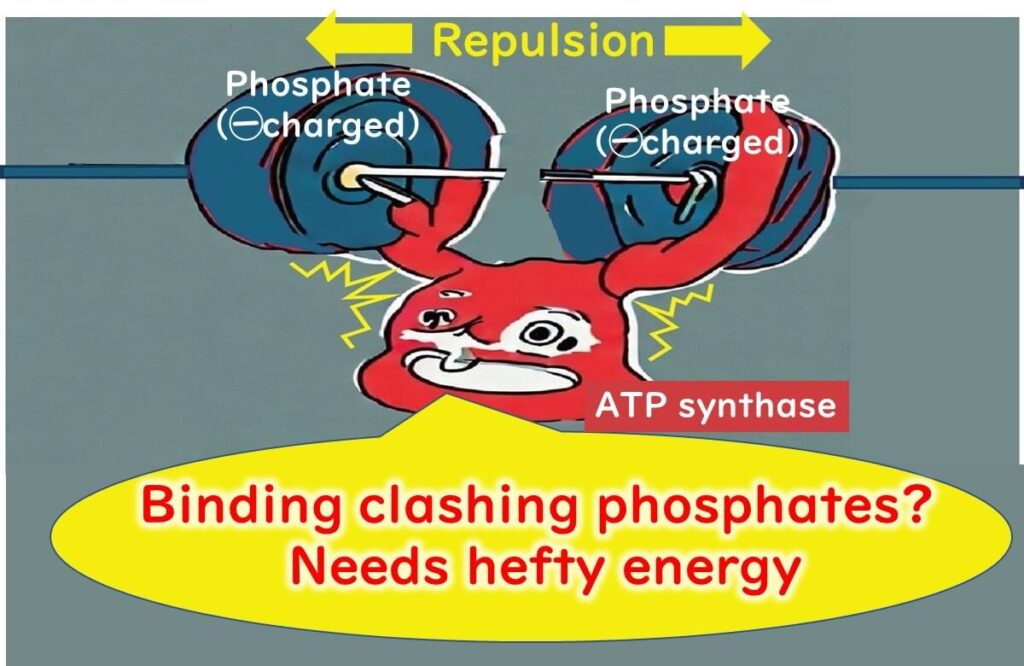
This property enables ATP to function as a universal energy carrier, playing an essential role in processes such as muscle contraction and biosynthesis within cells. Its versatility and energy density make it indispensable in the energy metabolism of living organisms.
ATP Measurement Methods
ATP measurement methods leverage the enzyme luciferase, famously known for its role in the bioluminescence of fireflies. To understand why luciferase is used, let’s first explore its characteristics and significance.
Bioluminescence in Fireflies and Marine Organisms
Fireflies produce light using luciferase, a process believed to offer ecological advantages, particularly in communication. Each species has a distinct bioluminescence pattern, enabling species-specific recognition and mate selection.
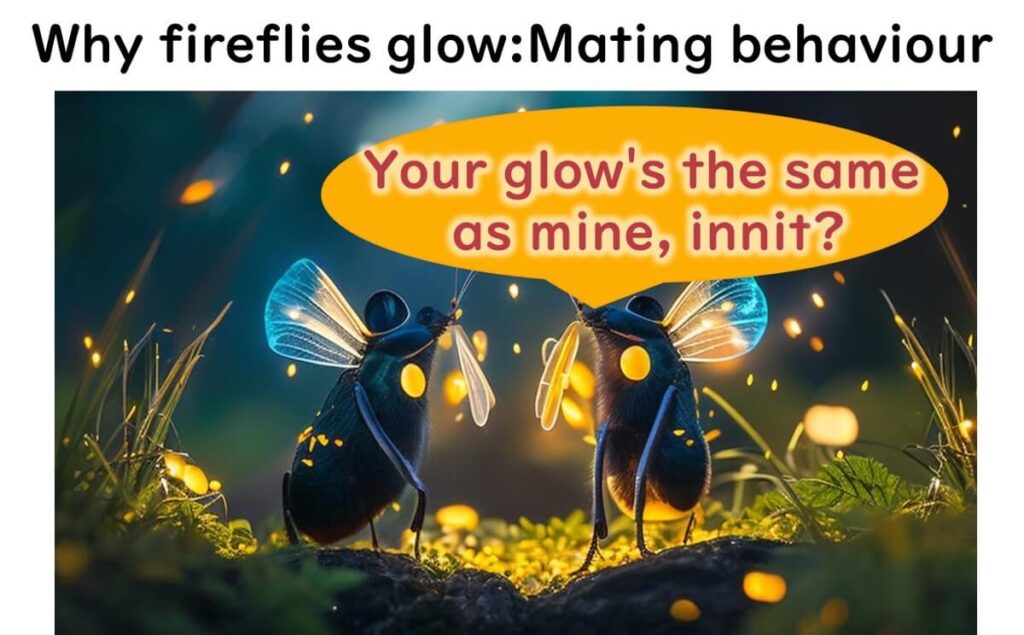
In the marine world, bioluminescent organisms use luciferase to thrive in dark environments. Their light serves various survival purposes, such as luring prey, evading predators, and signaling to others.
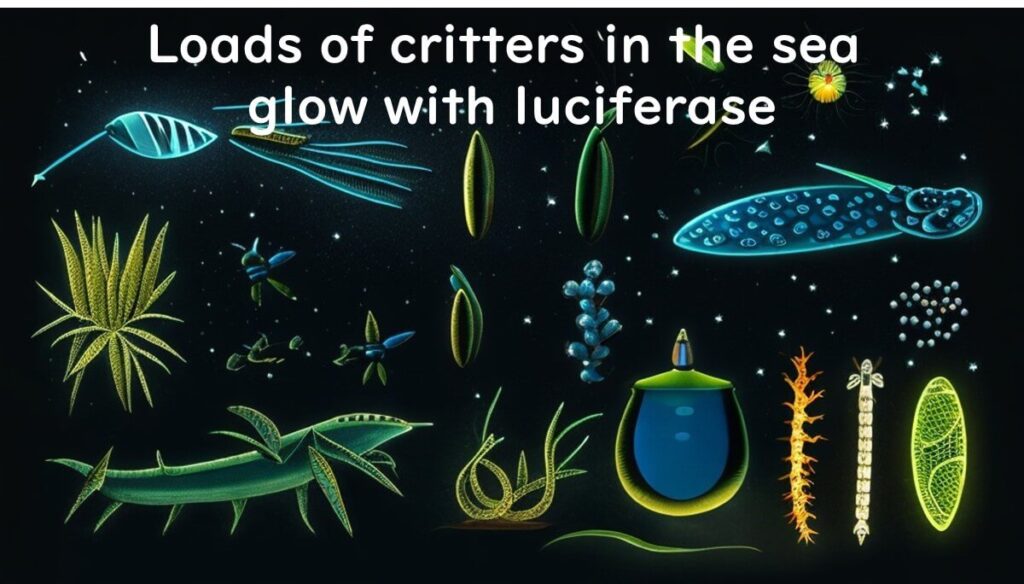
A notable example is bioluminescent plankton, which glows inside predators' digestive tracts, making them visible to larger predators. This defense mechanism often compels predators to expel the plankton, allowing them to escape harm.
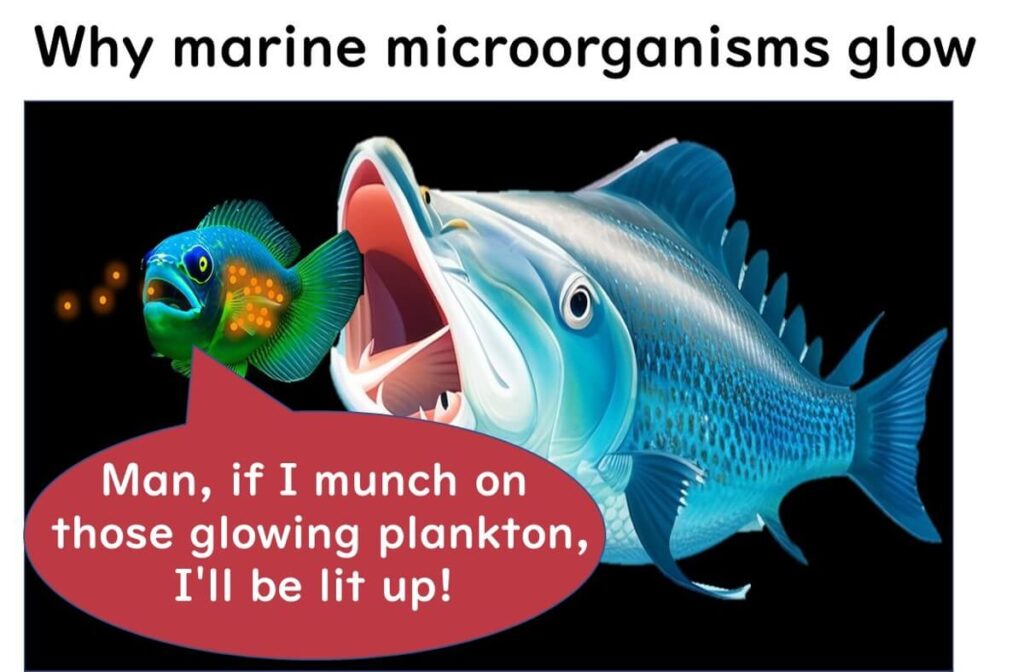
The Bioluminescent Reaction with Luciferase
Firefly bioluminescence is a chemical reaction catalyzed by luciferase, involving the following steps:
- The carboxyl group of luciferin reacts with ATP, forming a luciferyl AMP intermediate.
- This intermediate reacts with oxygen, generating an excited state of oxyluciferin.
- As oxyluciferin returns to its ground state, it emits a photon, producing light.
This reaction forms the foundation for ATP measurement, as the light emitted is proportional to the ATP concentration.
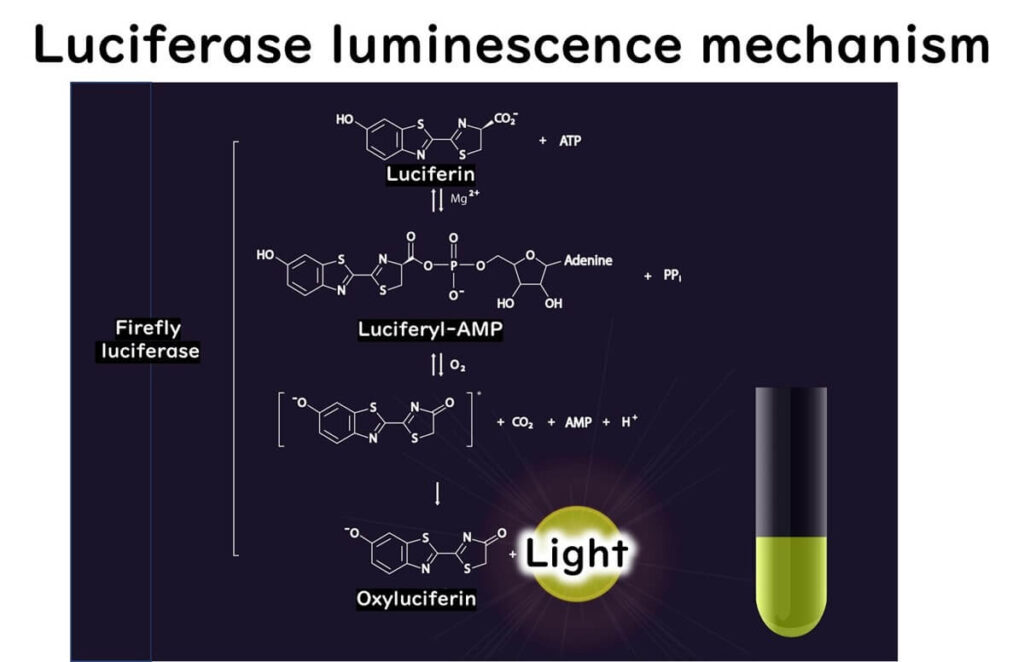
Application of Firefly Luciferase for ATP Quantification
The amount of light generated during the luciferase reaction directly correlates with ATP levels in biological samples. This relationship allows ATP quantification through light measurement.
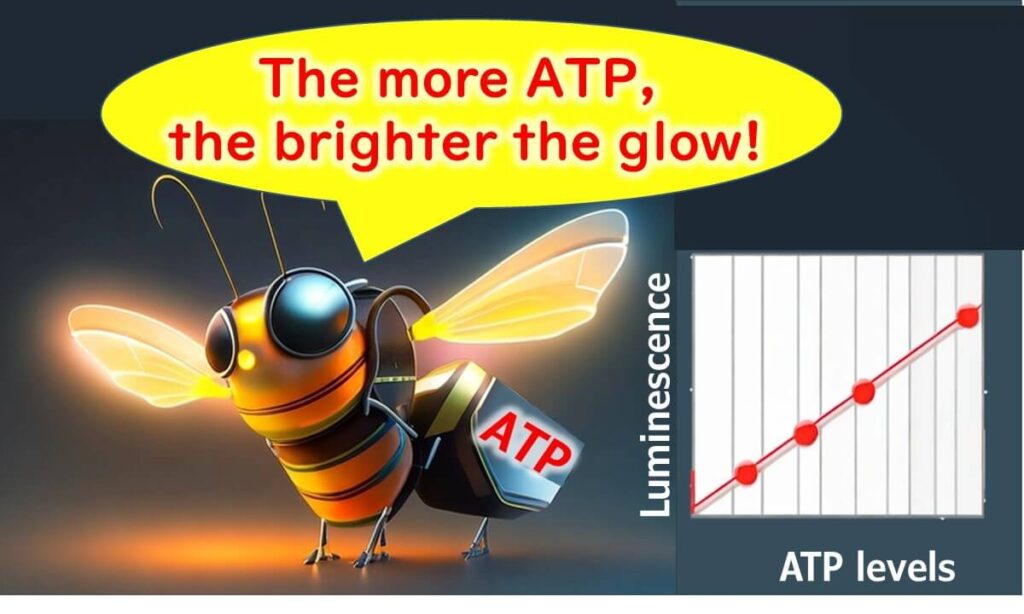
The first documented application of firefly luciferase for ATP measurement dates back to 1966, when Brewer and Knutsen explored ATP concentration in blood samples:
Brewer, G.J., Knutsen, C.A.
A technique for the processing of blood samples for subsequent assay of ATP, and an investigation of the method of standardization of the firefly-luciferase ATP assay
Clinica Chimica Acta 14(6), pp. 836-839( 1966 )
Since then, this method has become a cornerstone in biochemical research, finding applications across diverse scientific fields.
Mass Production of Luciferase through Genetically Modified E. coli
The methods for measuring ATP concentration using firefly luciferase have been well-established since the 1960s. However, the initial extraction and purification of luciferase directly from fireflies posed significant challenges, including high costs and limited stability, hindering its broader application.
A pivotal breakthrough occurred in the late 1980s with advancements in cloning techniques and recombinant expression, marking a new era in molecular biology. In 1988, Dr. Shigehiro Homma and his research team at Kikkoman Corporation in Japan successfully integrated the firefly luciferase gene into E. coli, enabling mass production of the enzyme.
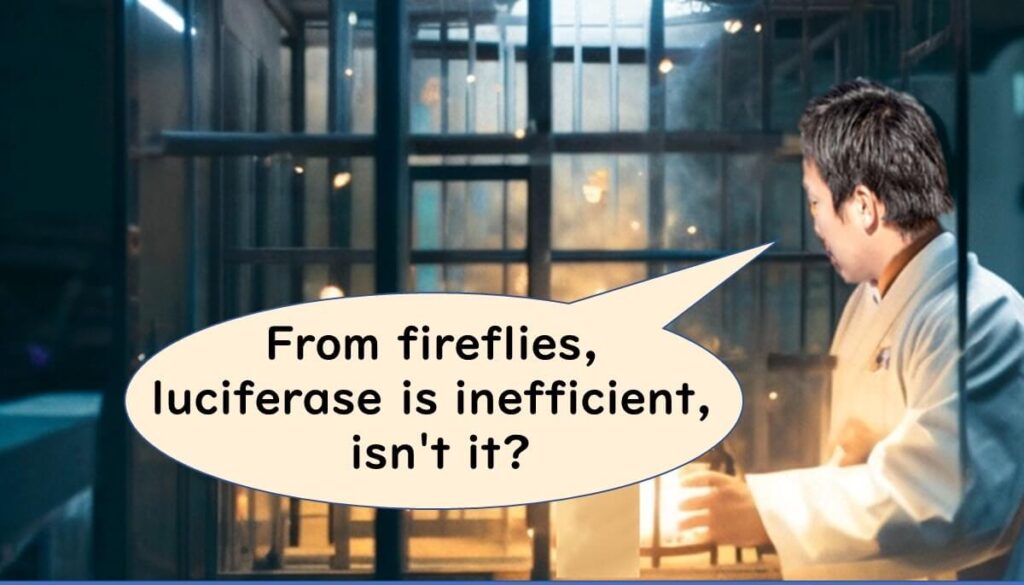
This innovation by Dr. Homma laid the groundwork for the widespread adoption of ATP measurement technology in the food industry. For more detailed information on these groundbreaking developments, refer to the relevant publications and resources.
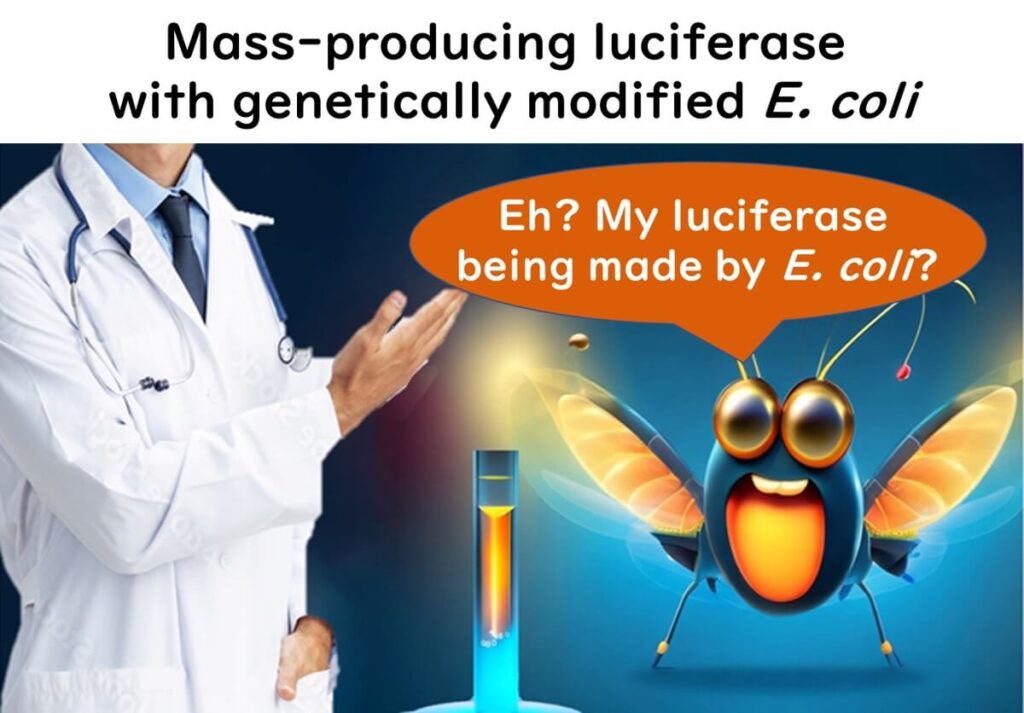
ATP Testing for Cleanliness Management in Food Factories
The mass production of luciferase has facilitated the widespread adoption of ATP testing in the food industry and other sectors.
Why is the ATP test crucial in food factories?
The ATP test stands out for its ability to provide rapid and straightforward cleanliness evaluations. Traditional microbial testing methods, while accurate, often involve lengthy and labor-intensive processes. The introduction of ATP testing has transformed this by enabling quick verification of cleaning effectiveness in food factories.
Initially, the ATP test was envisioned as a direct replacement for microbial testing. However, as ATP is also present in organic materials derived from food, it cannot exclusively indicate microbial contamination.
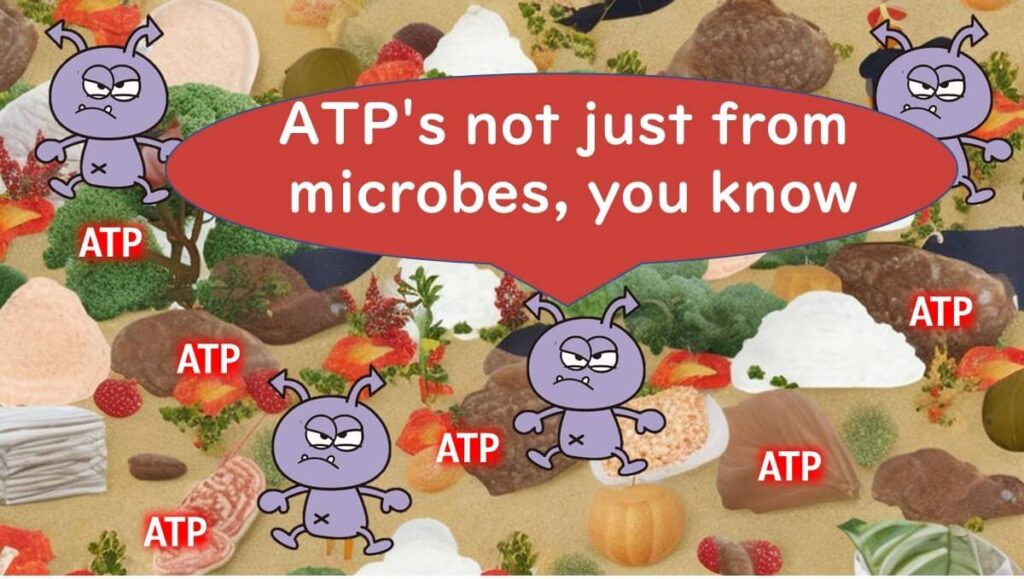
Today, the ATP test is primarily employed in food factories to measure the residual organic material left after cleaning. This practical application allows production lines to promptly verify whether proper cleaning protocols have been followed and adjust as necessary to maintain hygiene standards.
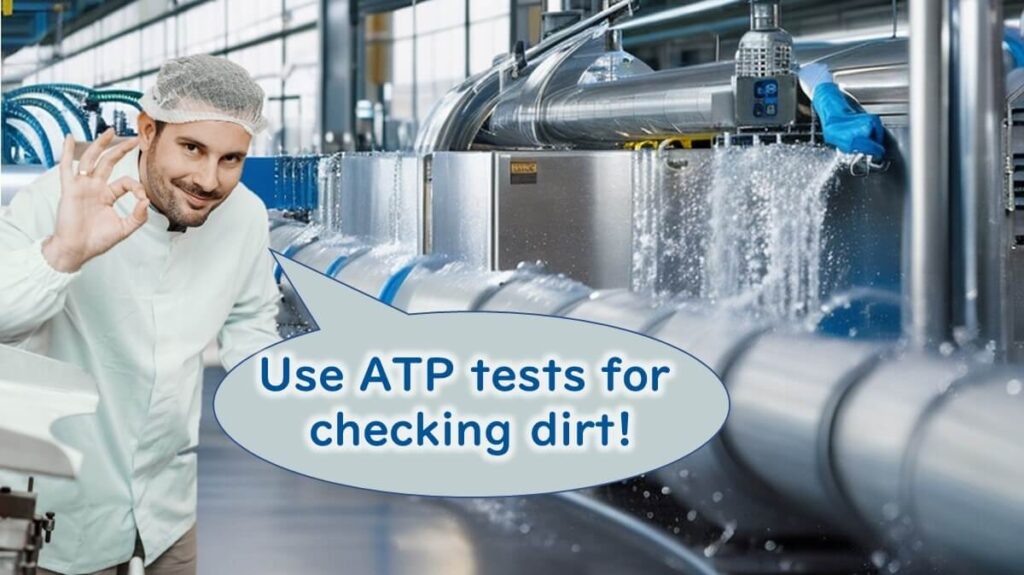
Measurement Procedure
Here is a concise outline of the general steps involved in conducting an ATP test. Please note that this blog serves as a conceptual guide rather than an experimental manual, aiming to clarify the broader aspects of the procedure.
❶Sample Collection
Samples are collected from the surfaces of equipment and the factory environment using swabs included with ATP testing kits. During the initial phase, sampling is conducted across the factory to establish baseline data. In subsequent phases, focus shifts to high-risk areas or points on production lines that are prone to contamination.
❷Sample Extraction
Collected samples are soaked in an extraction liquid designed to release ATP. This process is straightforward—insert the swab into the extraction liquid provided in the testing kit. Commercially available kits streamline this process for ease of use.
❸ATP Measurement
The extraction liquid is mixed with chemiluminescent reagents containing luciferin and luciferase. The light emitted from the reaction with ATP is measured, and its intensity correlates directly with the ATP concentration in the sample. Advanced kits combine sample extraction and reagent mixing into a single streamlined step.
❹Measurement Equipment
A luminometer is used to quantify ATP levels by detecting the light emitted during the reaction. The device converts light intensity into ATP concentration data. Some luminometers offer additional features, such as data recording and analysis, enhancing the usability for factory settings.
❺Result Interpretation
The luminometer results are compared against pre-established cleanliness standards tailored to each food factory’s specific conditions. This comparison determines whether cleaning protocols have achieved the desired level of hygiene.
Conclusion
Properly implementing ATP testing and understanding its limitations enables a more comprehensive cleanliness management approach in food factories. While the ATP test is not a direct substitute for microbial testing, it effectively highlights areas where organic residues remain, allowing for targeted cleaning and sterilization efforts. When combined with microbial testing where necessary, the ATP test becomes an integral part of an optimized hygiene management strategy.
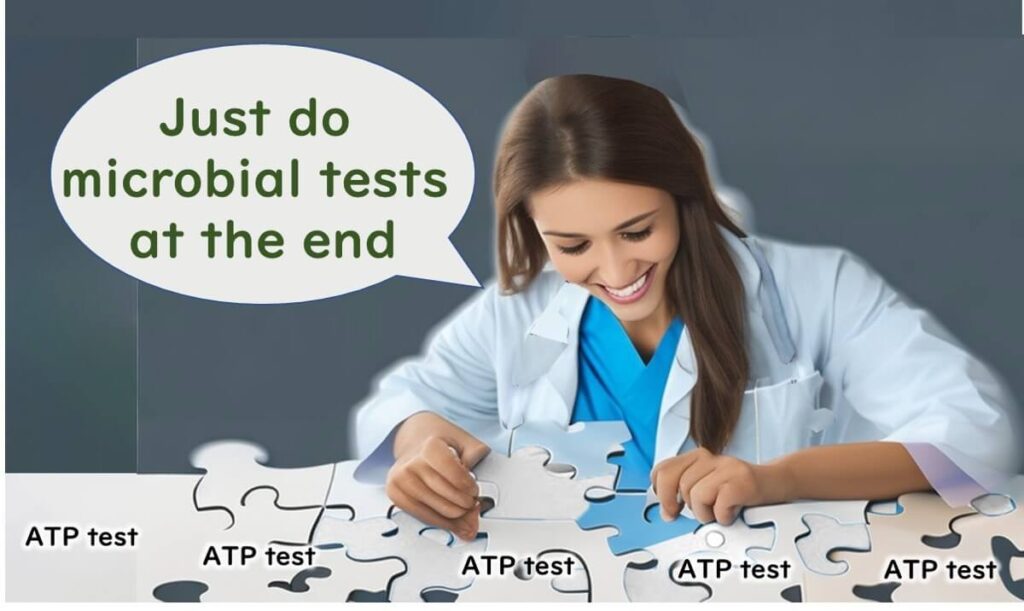