Understanding how microbes interact with temperature is essential for food safety. While many foodborne pathogens, such as mesophiles, thrive at moderate temperatures, there is a common misconception that psychrophiles—microbes that adapt to cold conditions—only grow in fridge-like environments. This article demystifies the growth behaviors of these temperature-sensitive bacteria, including the versatile Listeria monocytogenes, and explores the biological adaptations that allow certain microbes to thrive in colder climates. Strengthen your knowledge of microbiological principles critical to ensuring food safety.
Growth Temperatures of Foodborne Pathogens: Why Most Are Mesophilic
In food microbiology, understanding the relationship between microbial growth and temperature begins with recognizing that most bacteria responsible for infectious food poisoning cannot proliferate at refrigerator temperatures.
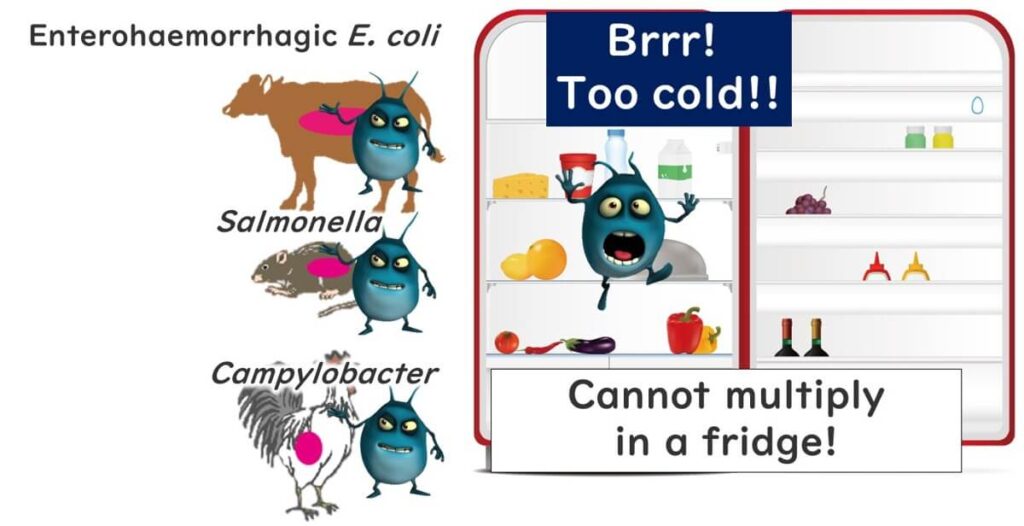
Why is this the case? These bacteria, which cause foodborne illnesses in humans, typically originate from the intestines of warm-blooded animals such as cattle, pigs, sheep, and birds. Their natural habitat—characterized by a warm, stable environment—does not require them to adapt to low temperatures. As a result, they are unable to multiply effectively in the colder conditions found in nature, particularly at temperatures below 10°C during winter.
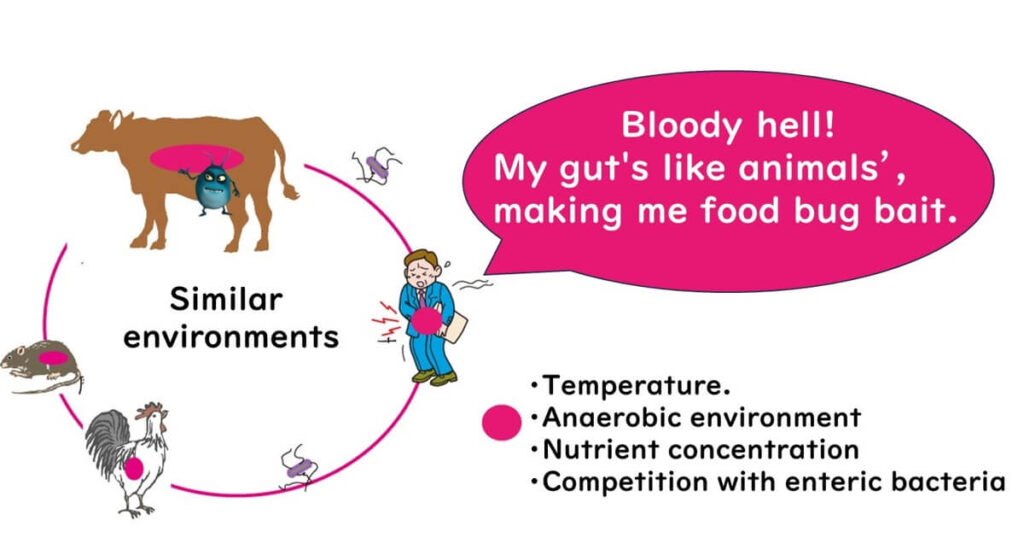
Psychrophiles Dominate in Nature: Adaptations to Cold Environments
In food microbiology, microbes that cannot grow at refrigerator temperatures are categorized as mesophiles. However, it is a common misconception among students of microbiology that mesophiles dominate in natural environments. In reality, they are exceptionally rare.
The majority of microbes in nature can thrive at refrigerator temperatures. This is especially evident in temperate regions, where winter temperatures often fall below 10°C. In such conditions, when animals like crows die or fish perish in ponds, these microbes decompose organic matter, playing a critical role in the elemental cycling that sustains Earth’s ecosystem. Without these cold-adapted bacteria, decomposition at low temperatures would be impossible.
Environmental microbes also naturally inhabit meat and vegetables. As a result, storing these foods in a refrigerator does not prevent spoilage—it merely slows it down. This spoilage is expected given the microbes' adaptation to cold environments. Conversely, as mentioned earlier, the bacteria that cause infectious food poisoning in humans cannot grow under typical refrigerator conditions.
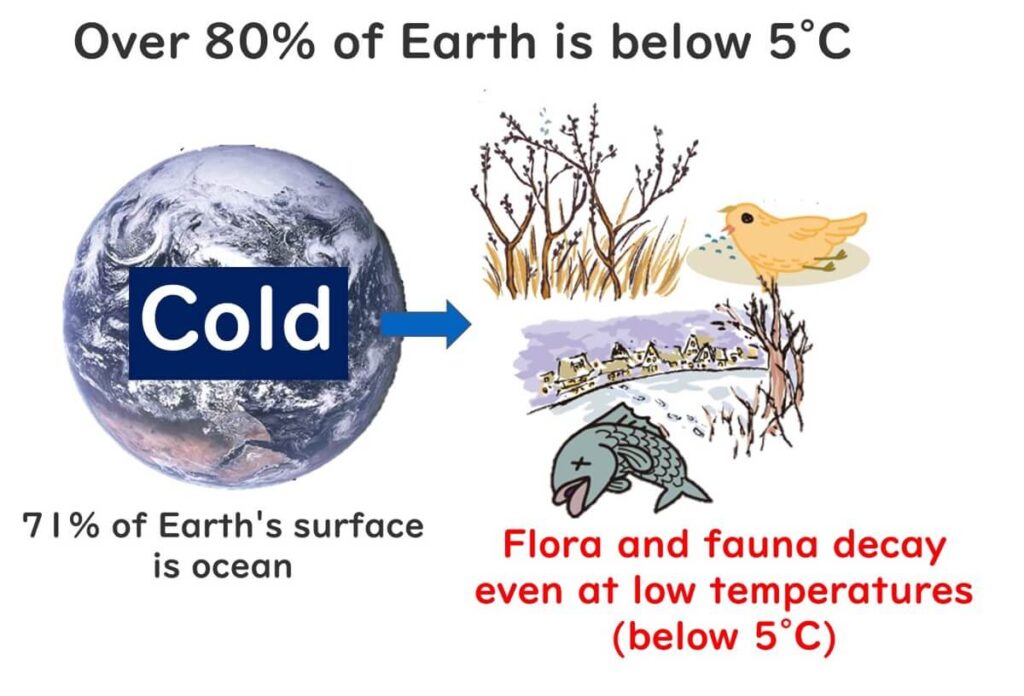
Debunking Misconceptions About the Minimum Growth Temperatures of Salmonella and E. coli
Understanding the relationship between microbial growth and temperature is fundamental in food microbiology. However, misconceptions often arise regarding whether bacteria responsible for infectious food poisoning can proliferate at refrigerator temperatures. This confusion is compounded by information in textbooks and online sources that list the minimum growth temperatures for foodborne pathogens below 10°C.
For instance, the U.S. FDA reports the minimum growth temperature for enterohemorrhagic E. coli as 6.5°C and for Salmonella as 5.2°C. At first glance, these figures might lead beginners in food microbiology to assume that these pathogens can actively multiply inside a refrigerator. This assumption, however, is incorrect.
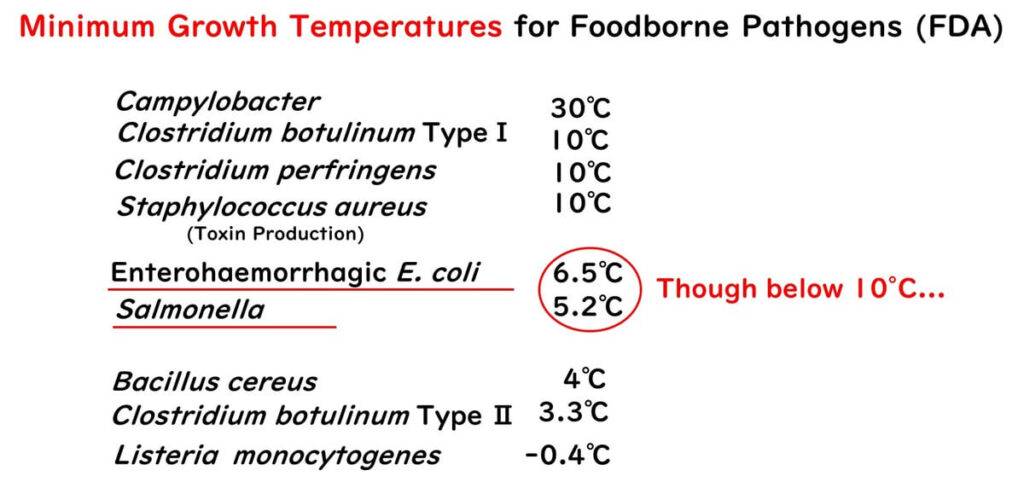
To clarify, let’s examine research data on the growth behaviors of enterohemorrhagic E. coli and psychrophilic spoilage bacteria in beef under various temperature conditions:
- At 4°C: Psychrophilic spoilage bacteria multiplied significantly after 72 hours, while there was no observable growth of enterohemorrhagic E. coli.
- At 7.2°C: Psychrophilic bacteria grew even more vigorously (data not shown), yet enterohemorrhagic E. coli still showed no growth after 72 hours.
- At 10°C: The upper limit of refrigerator temperature management, enterohemorrhagic E. coli exhibited minimal growth of approximately 0.5 log after 72 hours.
These findings underscore the importance of not relying solely on the numerical data for minimum growth temperatures. For example, assuming that "enterohemorrhagic E. coli can actively multiply in a refrigerator because its minimum growth temperature is 6.5°C" is a significant misunderstanding. The growth rate of psychrophilic spoilage bacteria at refrigerator temperatures far exceeds that of enterohemorrhagic E. coli. This distinction must be clearly understood and visualized to avoid misinterpretations.
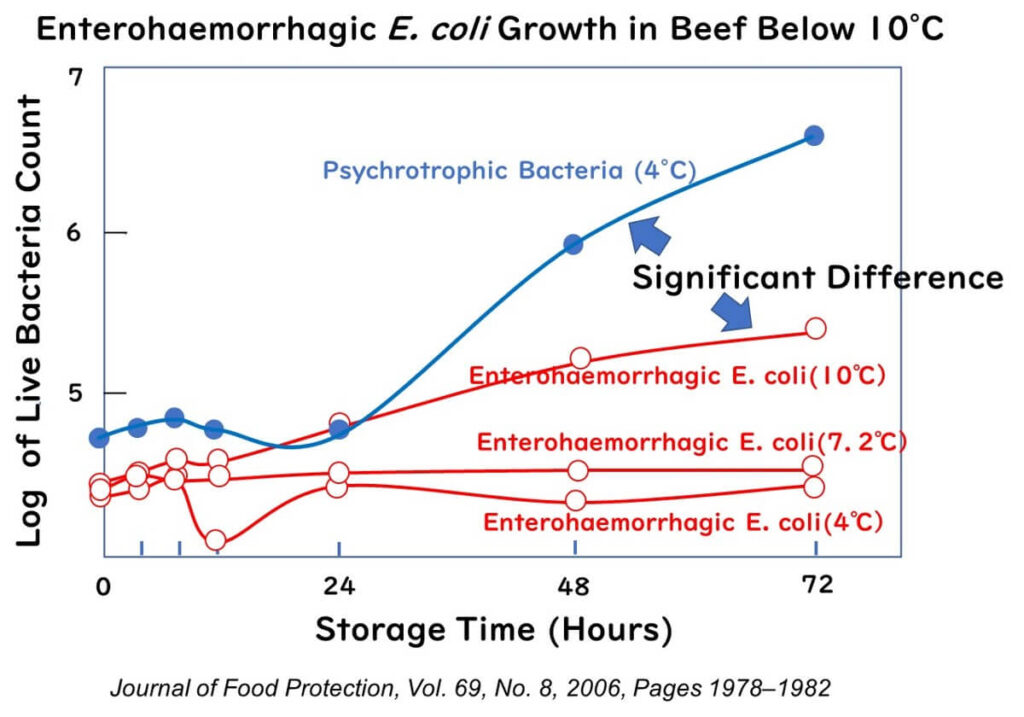
The graph illustrating this comparison was created by this blog author using data from the following paper:
Journal of Food Protection, Vol. 69, No. 8, 2006, Pages 1978–1981.
Journal of Food Protection, Vol. 69, No. 8, 2006, Pages 1978–1
How Psychrophiles Thrive: Mechanisms for Growth at Low Temperatures
To understand why psychrophiles can thrive in low-temperature environments, we must explore the biological mechanisms that enable their survival. While this concept is foundational in food microbiology, it provides valuable insights into various microbial phenomena.
Temperature and Microbial Activity
emperature fundamentally influences microbial life. At high temperatures, water molecules are highly active, while at low temperatures, their activity decreases. Microbial activity, in turn, depends on the behavior of cellular components like lipids, proteins, and carbohydrates.
Let’s focus on lipids, specifically fatty acids. Fatty acids can be categorized into two types:
- Unsaturated fatty acids, which have double bonds that create kinks in their structure. These kinks make the molecules scatter more easily, like restless children during a school assembly.
- Saturated fatty acids, which have no double bonds and align neatly, forming rigid, stable structures.
At high temperatures, active water molecules disturb even neatly aligned saturated fatty acids, causing slight instability. Unsaturated fatty acids, already structurally "restless," become even more unstable when exposed to this increased molecular activity.
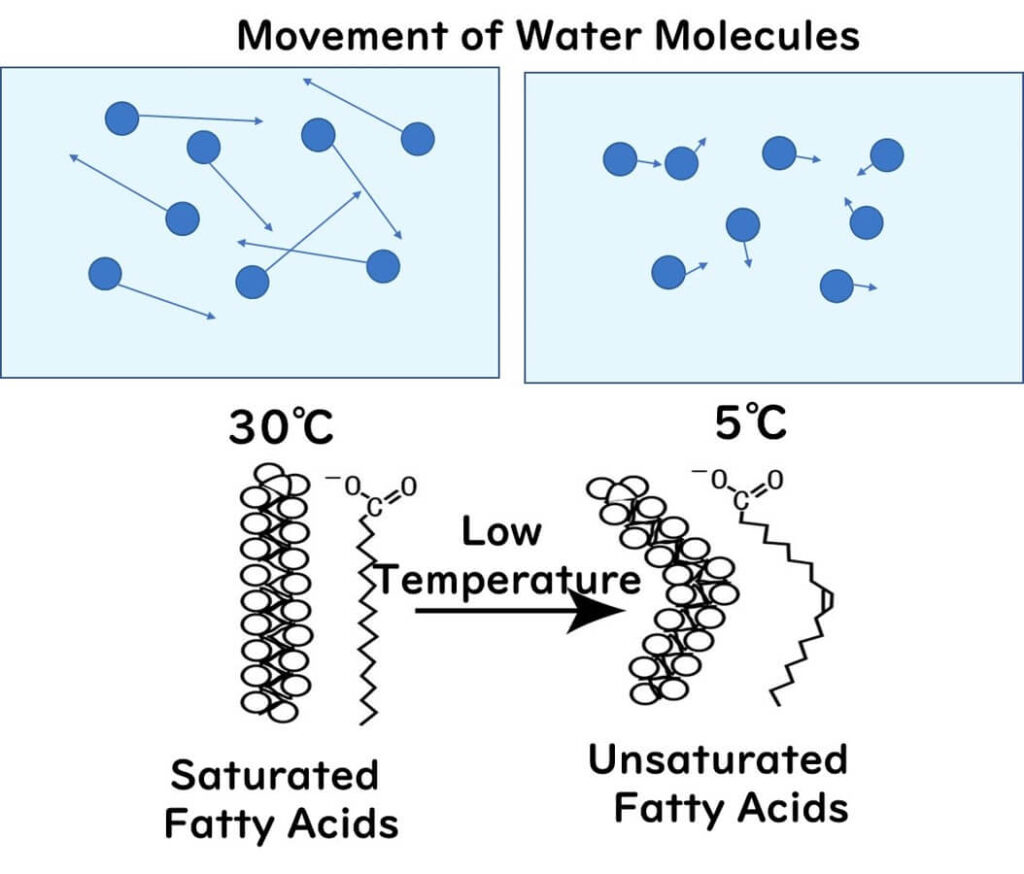
Conversely, at low temperatures:
- Unsaturated fatty acids remain flexible and functional, allowing cellular membranes to maintain fluidity.
- Saturated fatty acids become overly rigid and may solidify, impairing cellular processes.
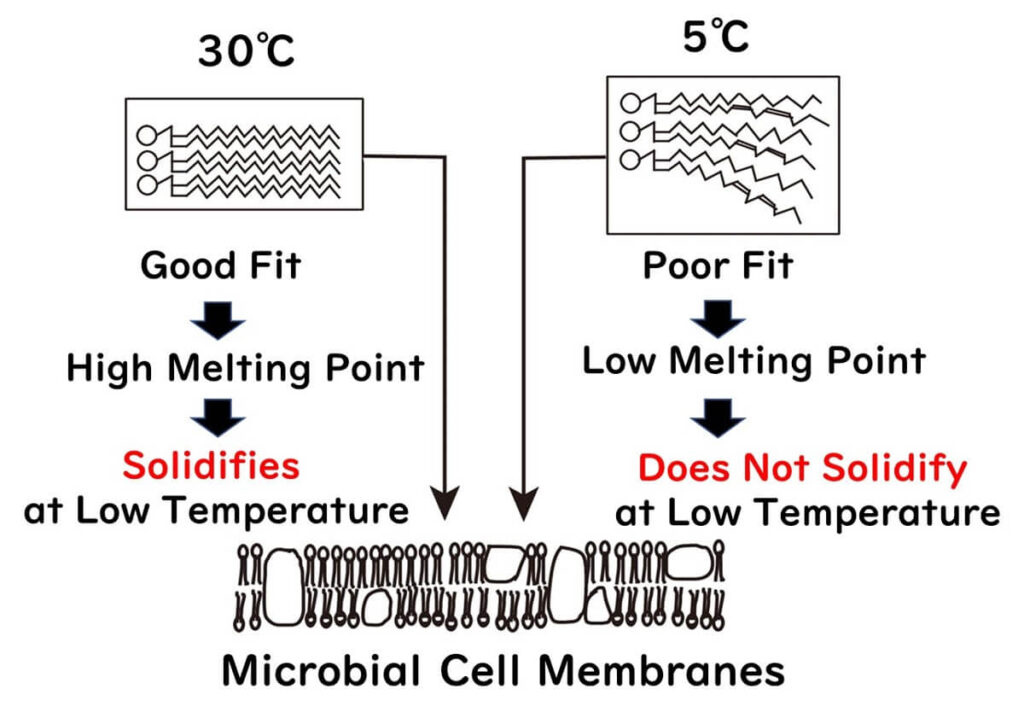
Practical Examples: Fatty Acids in Animals
This principle explains why:
Beef fat, composed mostly of saturated fatty acids, is solid at temperatures below 10°C but liquid at a cow’s body temperature of 37°C.
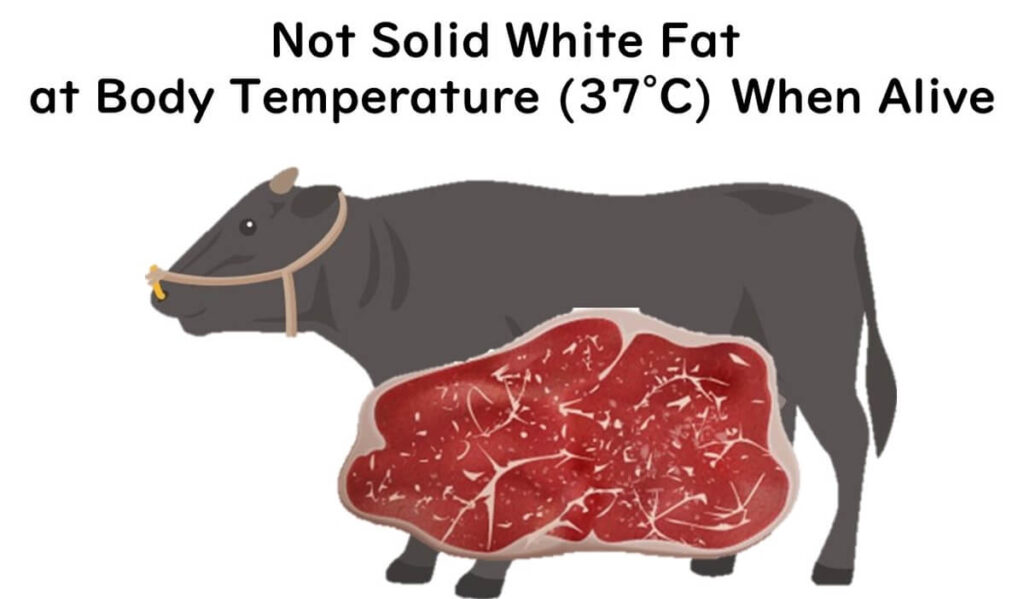
Fish fat, rich in unsaturated fatty acids, remains liquid even in refrigerated conditions. This adaptation allows fish to transition between warm surface waters (25°C) and cold deep-sea environments (below 10°C) without their fatty acids solidifying and disrupting mobility.
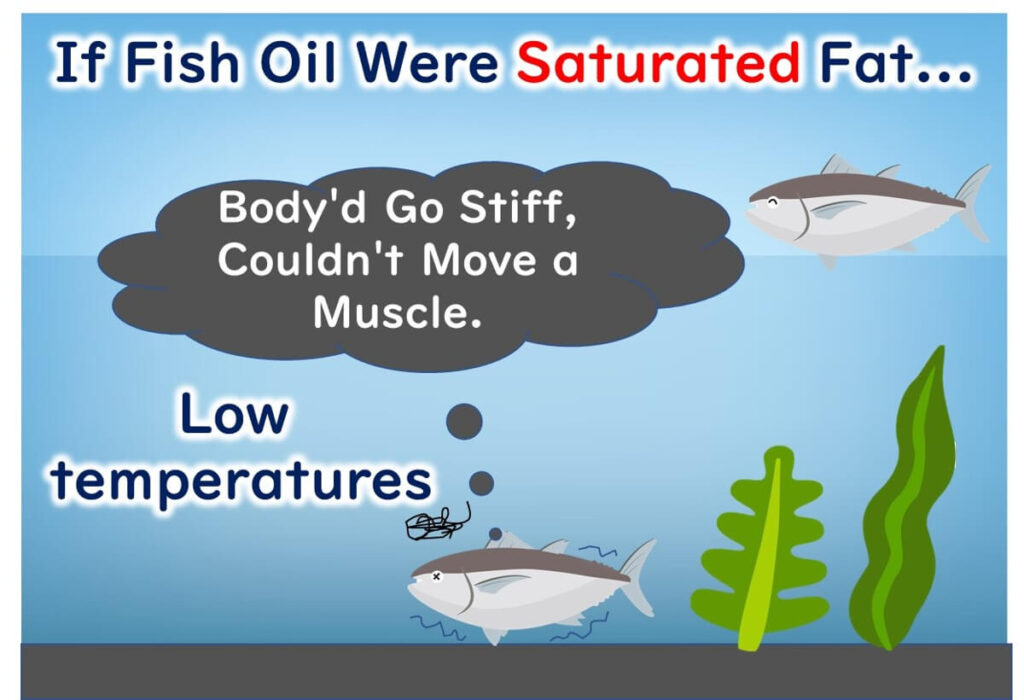
Proteins and Temperature Sensitivity
Proteins, composed of amino acids linked by peptide bonds, are also affected by temperature. While peptide bonds are stable, the hydrogen bonds that maintain protein structures are influenced by molecular activity:
At low temperatures, reduced molecular activity stabilizes protein structures. However, if temperatures drop too low, proteins lose the flexibility required for enzymatic activity.
At high temperatures, increased water molecule activity destabilizes hydrogen bonds, causing proteins to denature if fluctuations are excessive.
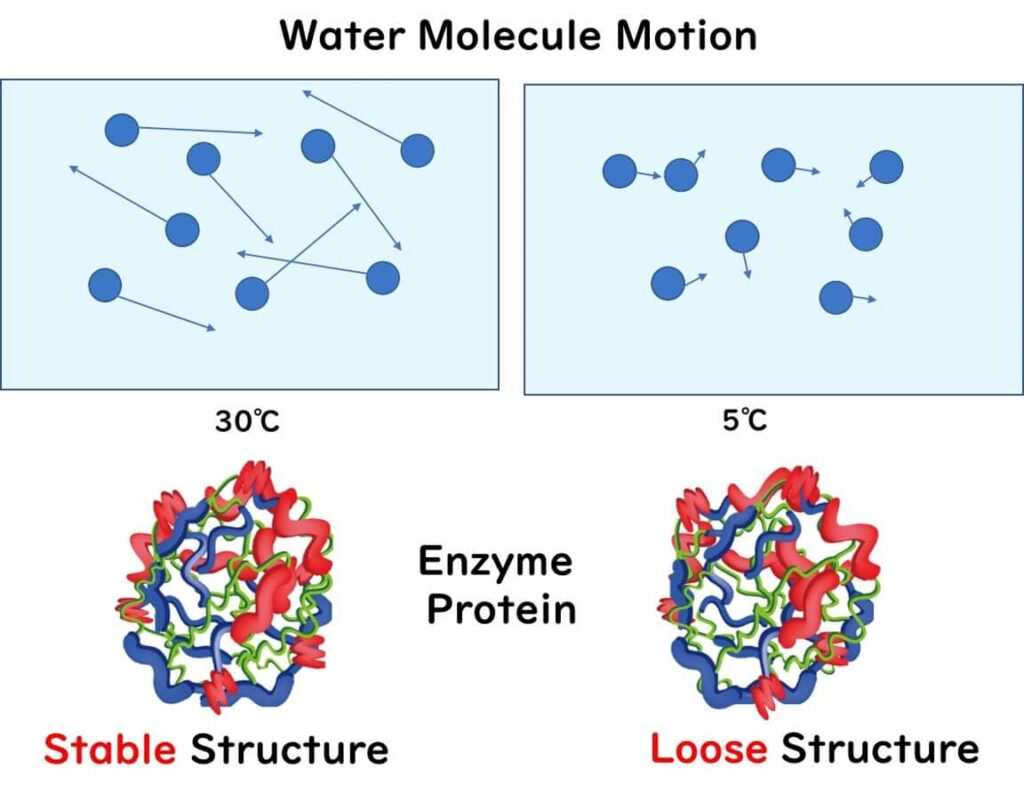
Psychrophiles’ Adaptations
Psychrophiles thrive at low temperatures due to their unique cellular adaptations:
- Their fatty acid composition is rich in unsaturated fatty acids, maintaining membrane fluidity in cold environments.
- Their enzyme proteins exhibit structural flexibility, allowing essential biochemical reactions to occur even under low-temperature conditions.
This intricate balance of lipid and protein adaptations enables psychrophiles to grow in environments where most other microbes cannot survive. These insights are crucial for understanding microbial behavior in cold food storage and preservation.
Why Microbes Capable of Growing Across Broad Temperature Ranges Are Rare
The relationship between microbial growth and temperature is influenced by key factors such as the proportions of saturated and unsaturated fatty acids in cell membranes and the amino acid composition of enzyme proteins, which determine their structural flexibility. For microbes to grow at low temperatures, their cellular components must undergo fundamental changes. However, these adaptations require specific genes that encode such modifications.
For instance, Listeria monocytogenes is capable of proliferating at both mammalian body temperatures and the cooler conditions of a refrigerator. In contrast, most bacteria causing infectious food poisoning are adapted to thrive near mammalian body temperatures and cannot multiply in refrigerator environments.
Why Don’t All Microbes Have Broad Temperature Adaptability?
At first glance, it might seem advantageous for microbes to possess the ability to grow across a wide range of temperatures, as Listeria does. However, this capability comes with trade-offs. For Listeria to adapt to such conditions, it must carry genes that enable changes in its fatty acid and protein compositions. This means Listeria has a more complex genome compared to microbes that grow only within a mesophilic range.
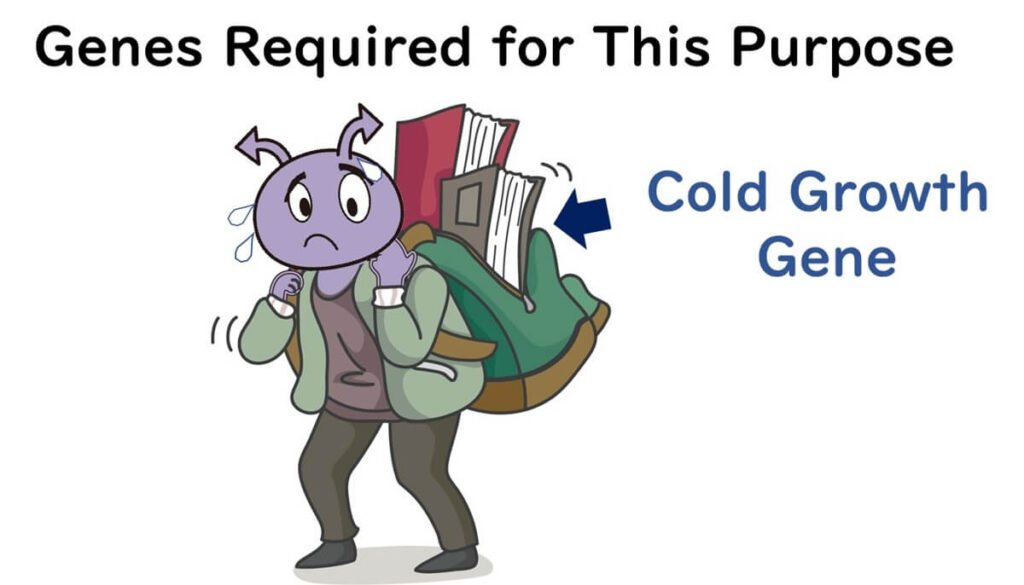
While having more genes may appear beneficial, microbial life is highly specialized. Microbes are often adapted to thrive in specific niches rather than across diverse environments. For instance, in the case of Escherichia coli residing in mammalian intestines, genes enabling growth at low temperatures would be unnecessary. Carrying such superfluous genes could slow down the division rate, making the bacteria less competitive in its primary habitat.
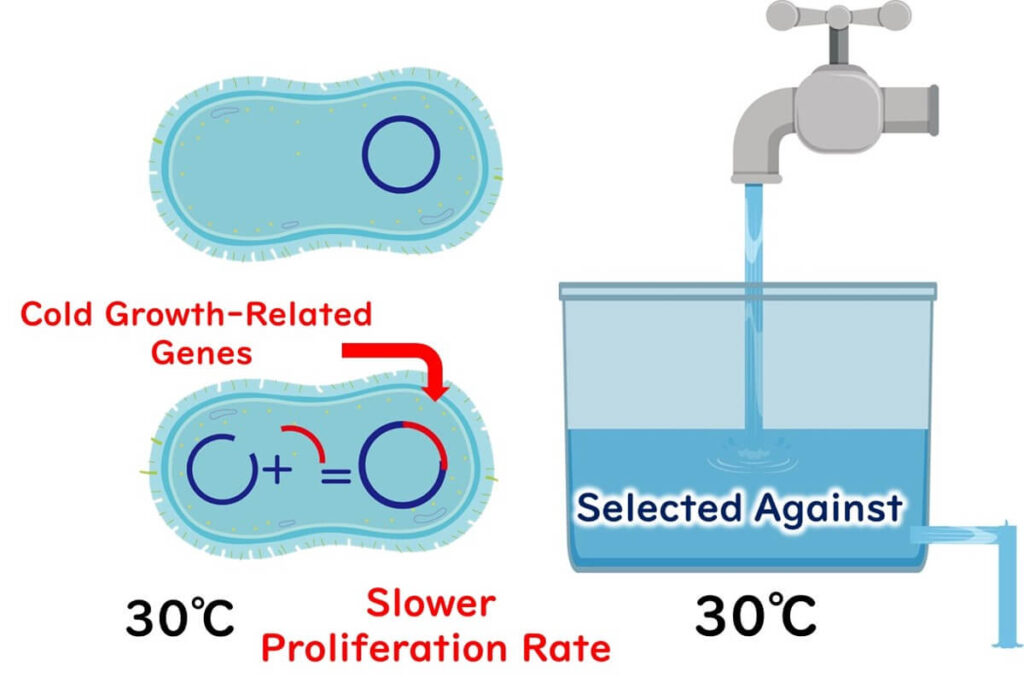
Specialization vs. Versatility
In nature, efficiency is key. Microbes typically carry only the genes required for optimal growth within their specific environment. This specialization can be likened to professional sports:
- A baseball player focuses on pitching or batting, not both.
- A football player excels as a goalkeeper or a forward, but rarely both.
Similarly, bacteria do not waste resources maintaining unnecessary capabilities. The competition within microbial ecosystems is intense, leaving little room for "all-rounders." This explains why microbes like Listeria, capable of growing across a broad temperature range, are relatively rare.
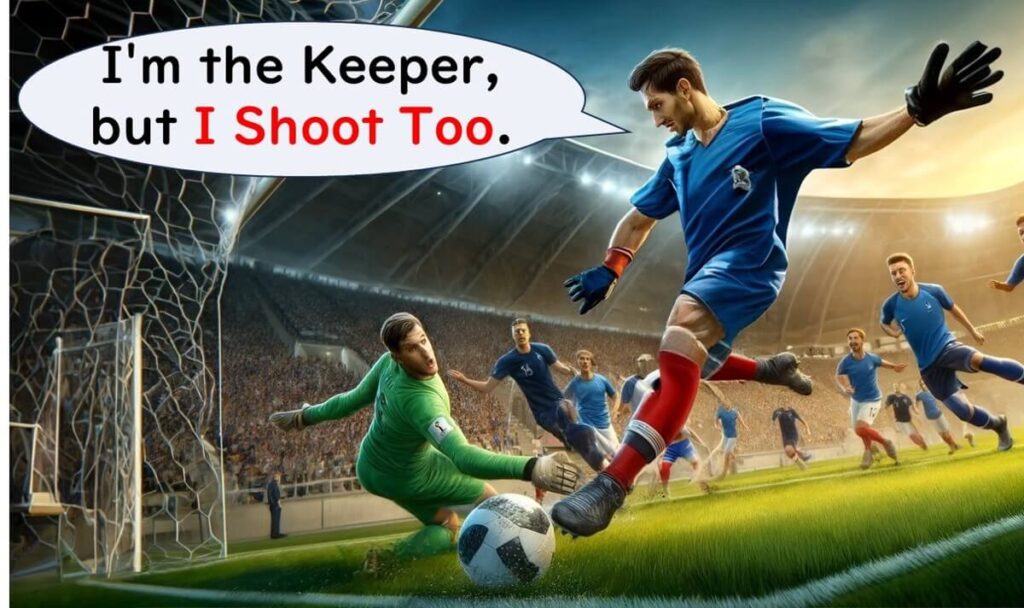
Methods for Testing Psychrophilic Bacteria
Standard Methods for General Viable Counts
In Japan, food hygiene inspections typically use the AOAC method, which involves incubation at 35°C for 48 hours. Globally, two main methods are recognized:
- American AOAC method: 35°C for 48 hours (equivalent to Japan's official method).
- European ISO method: 30°C for 72 hours.
Each method has its pros and cons. For example:
Speed is crucial for routine testing, giving the AOAC method an edge for rapid results.
Lower temperatures and longer incubation times (ISO method) allow for higher bacterial counts, with studies reporting that the ISO method detects 0.18 log more bacteria than the AOAC method.
Reference
https://sfamjournals.onlinelibrary.wiley.com/doi/full/10.1111/jam.13227
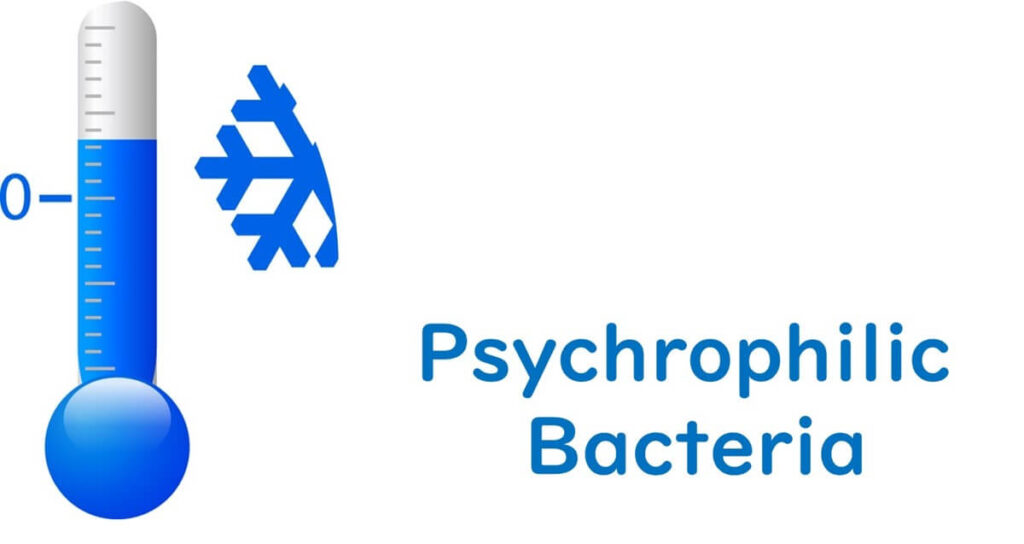
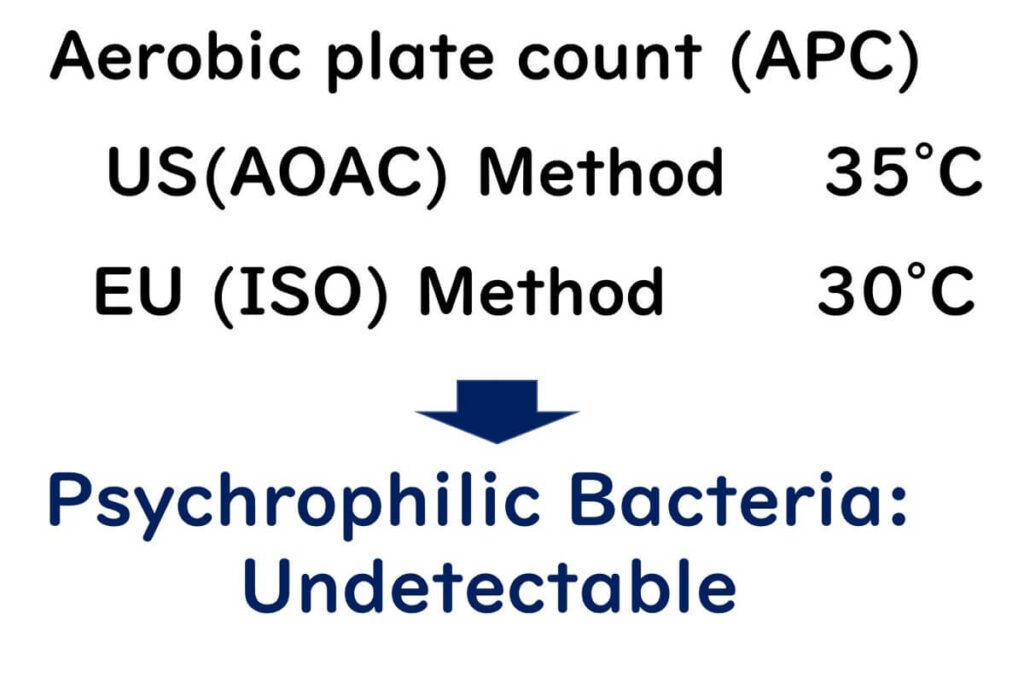
Measuring Psychrophilic Bacteria: The Challenges
The standard methods mentioned above are not suited for psychrophilic bacteria, which thrive at much lower temperatures. This is especially relevant for bacteria commonly found on seafood and vegetables in temperate or cold regions. For accurate detection, the cultivation temperature must reflect the growth range of psychrophiles.
By definition, psychrophiles are not strictly classified by taxonomy but are broadly defined as bacteria capable of proliferating at low temperatures during food preservation. This lack of consensus on definitions has caused confusion:
- Some define psychrophiles as "obligate psychrophiles" that grow only at low temperatures (optimal growth between 10°C and 20°C or lower).
- Others consider bacteria with an optimal growth temperature below 20°C as psychrophiles.
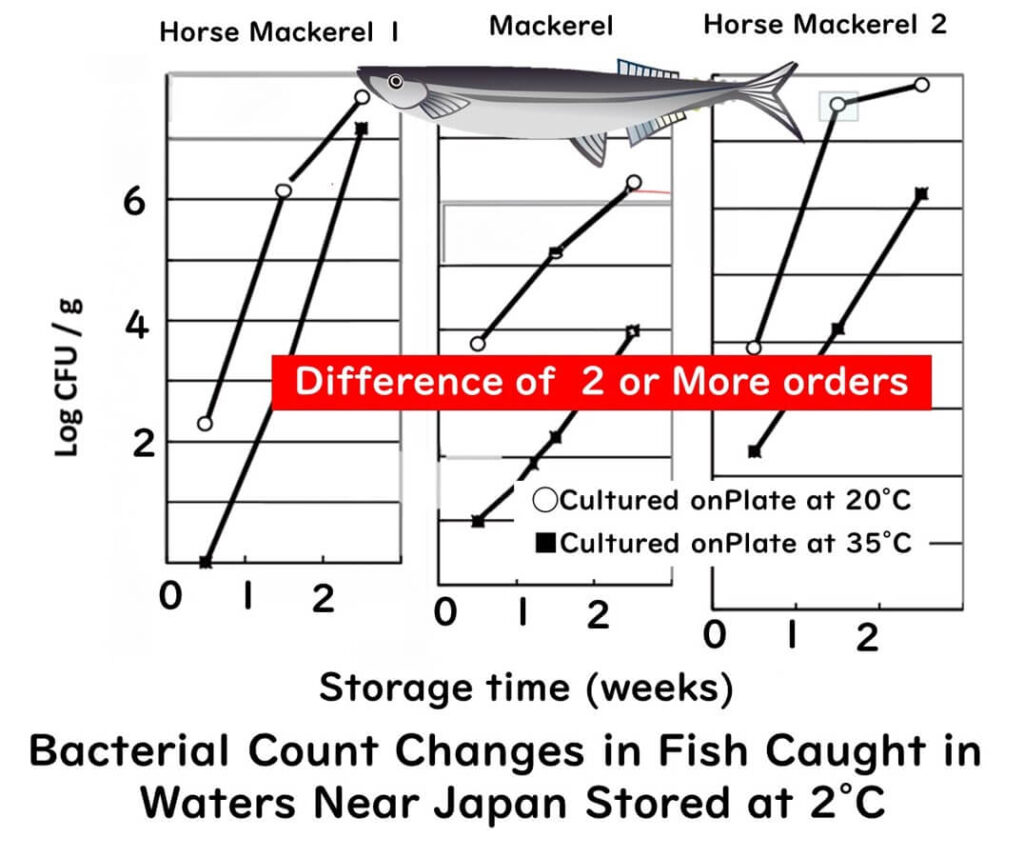
The Impact of Incubation Temperature on Detection Accuracy
Many bacteria encountered in food handling environments cannot grow at 30°C. For example:
- When measuring bacteria on fish, the AOAC method (35°C for 48 hours) significantly underestimates actual bacterial counts.
- If not incubated below 25°C, bacterial counts may be underestimated by up to two orders of magnitude, potentially missing 99.9% of the bacterial flora.
Common psychrophilic bacteria found on fish, such as Alteromonas, Psychrobacter, and Shewanella, often cannot grow above 30°C. This highlights the importance of using appropriate temperatures for microbial analysis.
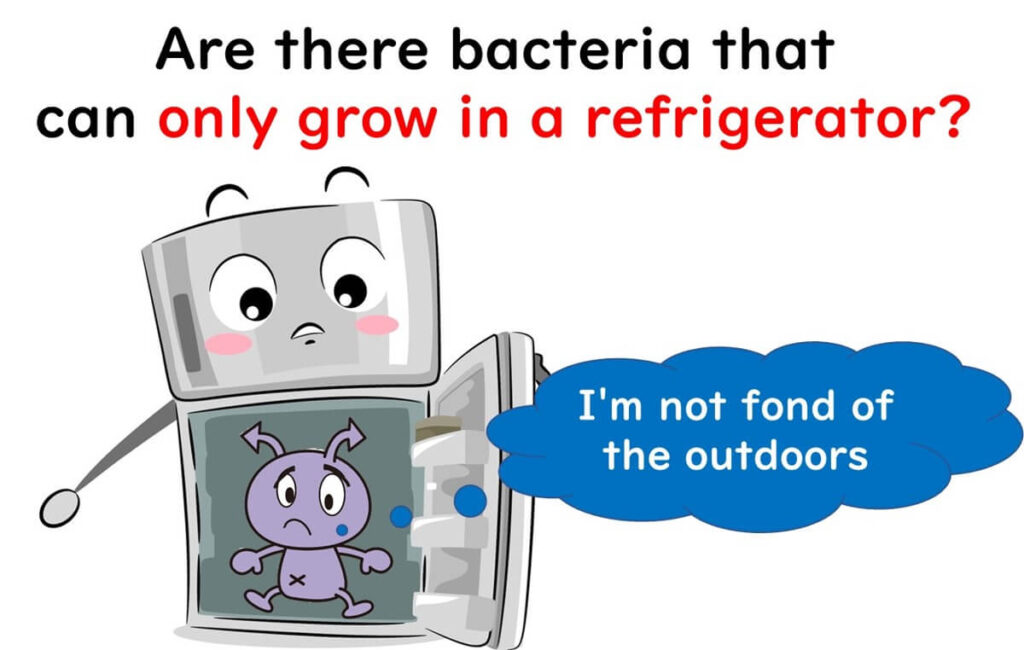
Historical Context: Discovering True Psychrophiles
Research on psychrophiles dates back to the 1960s, when global efforts sought to identify bacteria that could grow at low temperatures. Initial studies were disappointing, as many isolates grew at 25°C or 20°C, failing to meet the criteria for true psychrophiles. It was not until researchers like Dr. Richard Morita explored extreme environments, such as Antarctica, that true psychrophiles were discovered, with maximum growth temperatures as low as 20°C.
Reference
https://pubmed.ncbi.nlm.nih.gov/1095004/
Practical Insights for Testing Psychrophilic Bacteria
In routine food testing, bacteria incapable of growing at 30°C are frequently encountered. However, those unable to grow below 25°C are less common. For products like fresh fish, where psychrophiles are expected, cultivating samples at 25°C is often practical and effective. This approach avoids underestimating bacterial counts while maintaining efficiency.
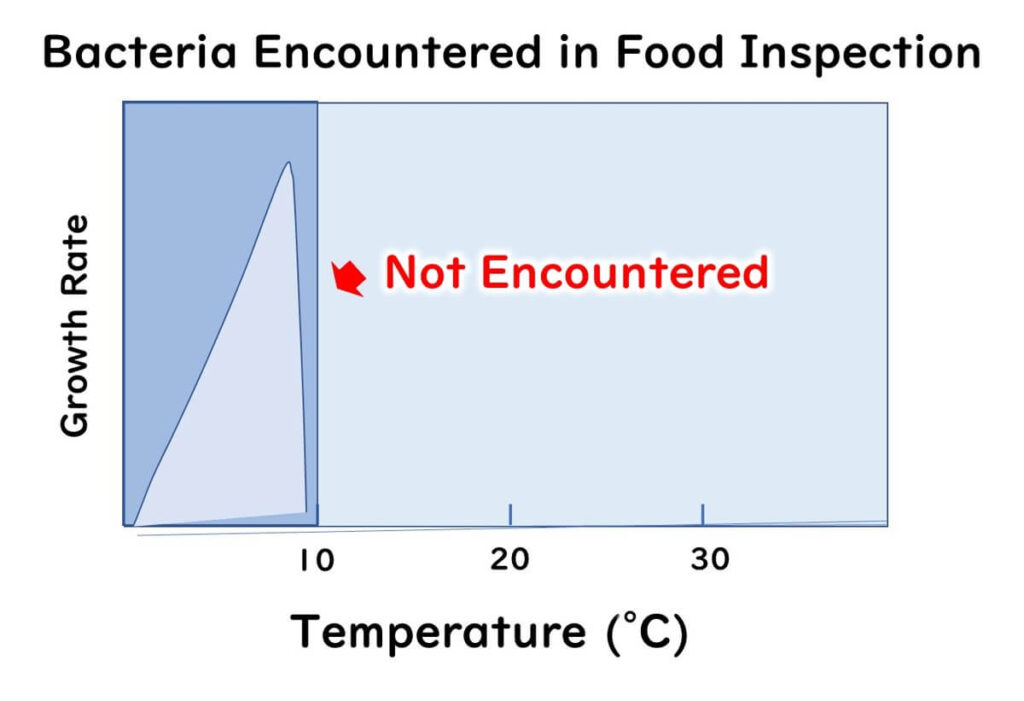
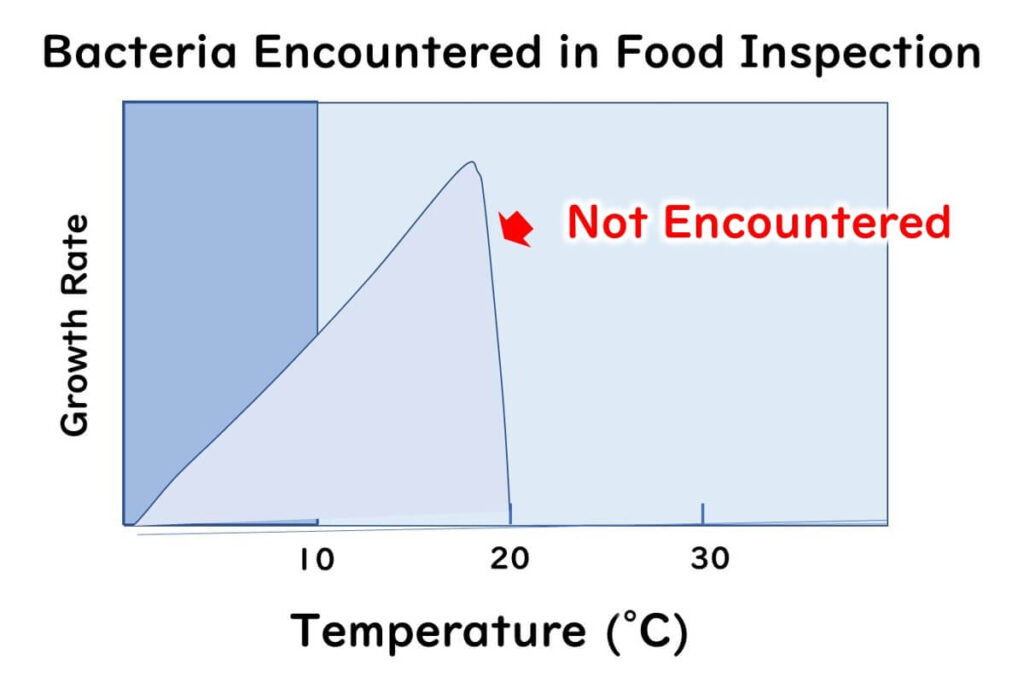
Importantly, this article clarifies the misconception that psychrophilic bacteria grow only at refrigerator temperatures. While 30°C is a critical threshold for many low-temperature bacteria, their growth characteristics must be accurately understood to ensure reliable testing.
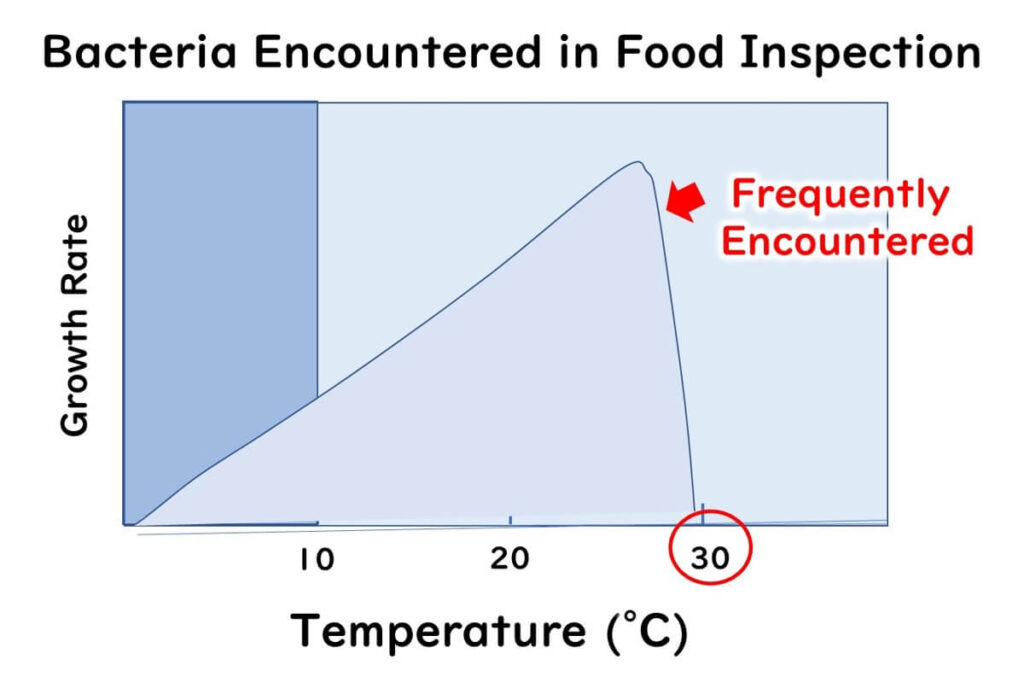
Understanding the Growth Rate of Microbes at Low Temperatures
Discussions about psychrophiles often raise concerns among young quality control professionals in private companies, particularly regarding foodborne pathogens that can grow at low temperatures. A common misconception is that these pathogens, such as Listeria monocytogenes, Bacillus cereus, and Clostridium botulinum type II, can proliferate rapidly under cold conditions, posing significant risks. While it is true that these low-temperature-growing pathogens require careful management, their growth rates are limited by biological constraints.
The Role of Enzyme Kinetics in Growth Rate
The growth of psychrophilic foodborne pathogens is fundamentally governed by the kinetics of enzyme reactions. As temperature decreases, the molecular activity required for enzymatic processes slows down, resulting in reduced proliferation rates. This means that while these bacteria can grow at low temperatures, their growth is far from rapid.
For instance:
- Food poisoning caused by Bacillus cereus in improperly stored food is often attributed to extended storage durations at low temperatures rather than the bacteria's intrinsic ability to grow quickly under such conditions.
- More frequently, foodborne illness arises from temperature management failures rather than the exceptional growth capabilities of psychrophilic pathogens.
Misconceptions About Low-Temperature Proliferation
It’s crucial to dispel the notion that low-temperature pathogens can multiply as quickly as mesophilic bacteria under optimal conditions. Effective temperature control is a critical preventative measure in food safety. Overestimating the growth rates of psychrophilic bacteria may lead to misplaced focus, detracting from addressing actual temperature management challenges.
Practical Implications for Food Safety Practices
Quality control professionals must recognize that while psychrophilic pathogens grow in colder environments, their growth rates remain limited. Effective refrigeration, proper storage durations, and strict adherence to temperature controls are essential to preventing foodborne illnesses. By focusing on these preventative measures, food safety practices can be aligned with a more accurate understanding of microbial behavior under varying temperature conditions.
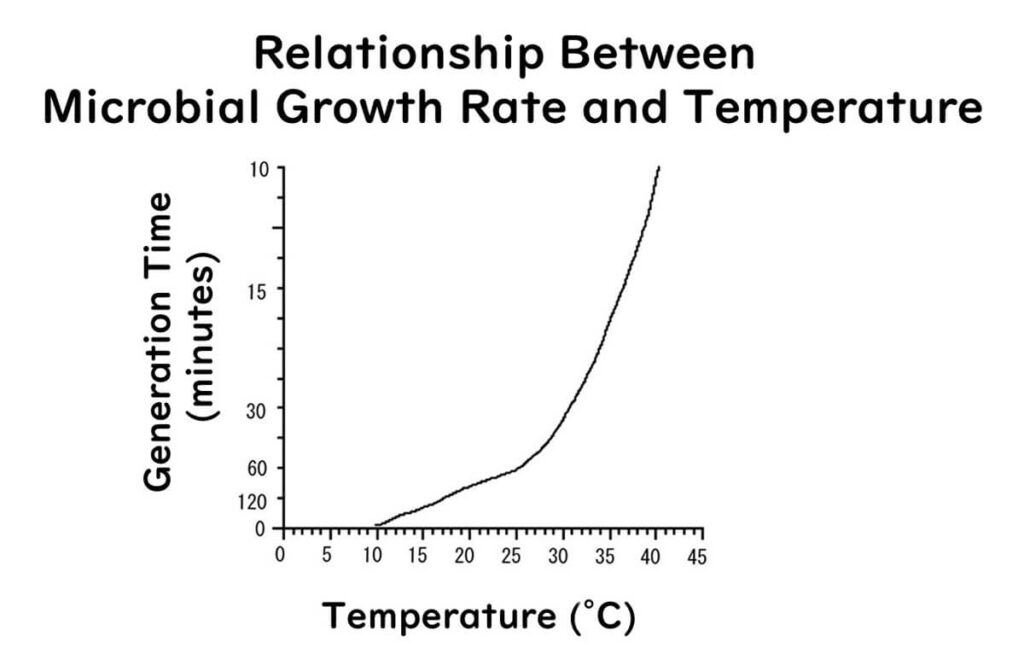
The relationship between temperature and microbial growth is illustrated in a composite view of actual growth rates for multiple bacteria, including E. coli and Staphylococcus aureus. This data, adapted from McMeekin (1996), emphasizes the significant decline in growth rates as temperatures drop.
Int J Food Microbiol Nov;33(1):65-83.(1996)