High-Pressure Processing (HPP), also referred to as High Hydrostatic Pressure (HHP) or Ultra High Pressure (UHP), is a revolutionary non-thermal food preservation technology designed to inactivate foodborne pathogens and spoilage bacteria. Unlike traditional thermal processing, HPP minimizes negative effects on the taste, texture, appearance, and nutritional value of foods, making it a preferred alternative for maintaining food quality. In this article, we delve into the fundamentals of HPP, focusing on its application in microbial sterilization and its advantages over conventional sterilization methods.
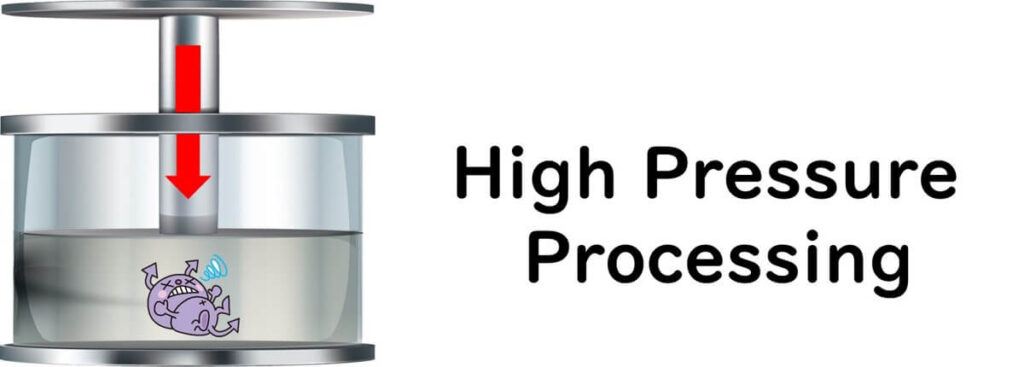
Sterilization of Food: High-Pressure Processing as a Modern Alternative
Sterilization of food is traditionally achieved through thermal processing, with pasteurization being a cornerstone technique. However, thermal methods often compromise the flavor, texture, and nutritional quality of food. High-Pressure Processing (HPP) offers a promising alternative, overcoming these drawbacks while maintaining food quality.
Overview of the Technology
High-Pressure Processing (HPP) is a non-thermal treatment that applies uniform isotropic pressure to both solid and liquid foods. The pressure is distributed quickly and evenly, ensuring all parts of the food are exposed simultaneously.
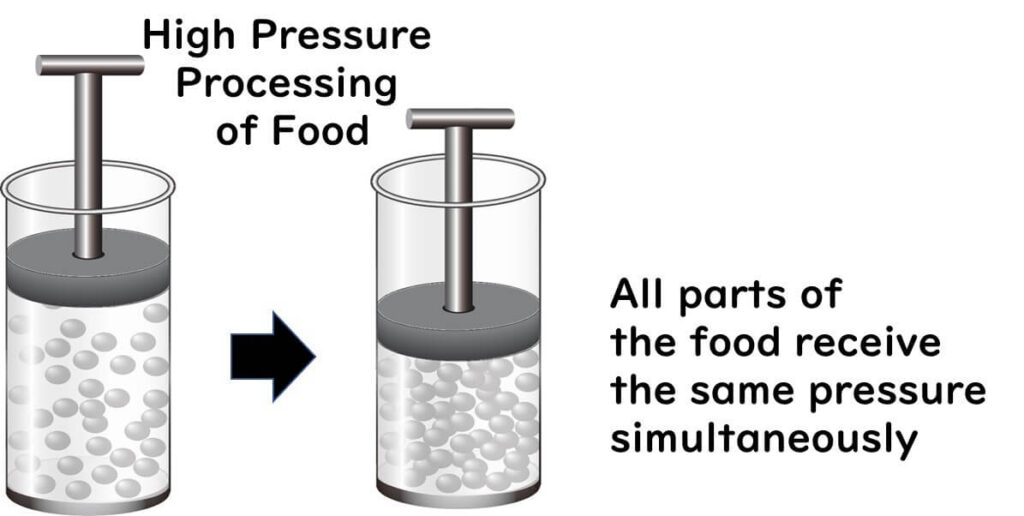
Typically, HPP is conducted at refrigeration or mild processing temperatures (below 45°C) with pressures ranging from 400 to 600 MPa. The hold times commonly vary between 1.5 to 6 minutes, depending on the food product and target microorganisms.
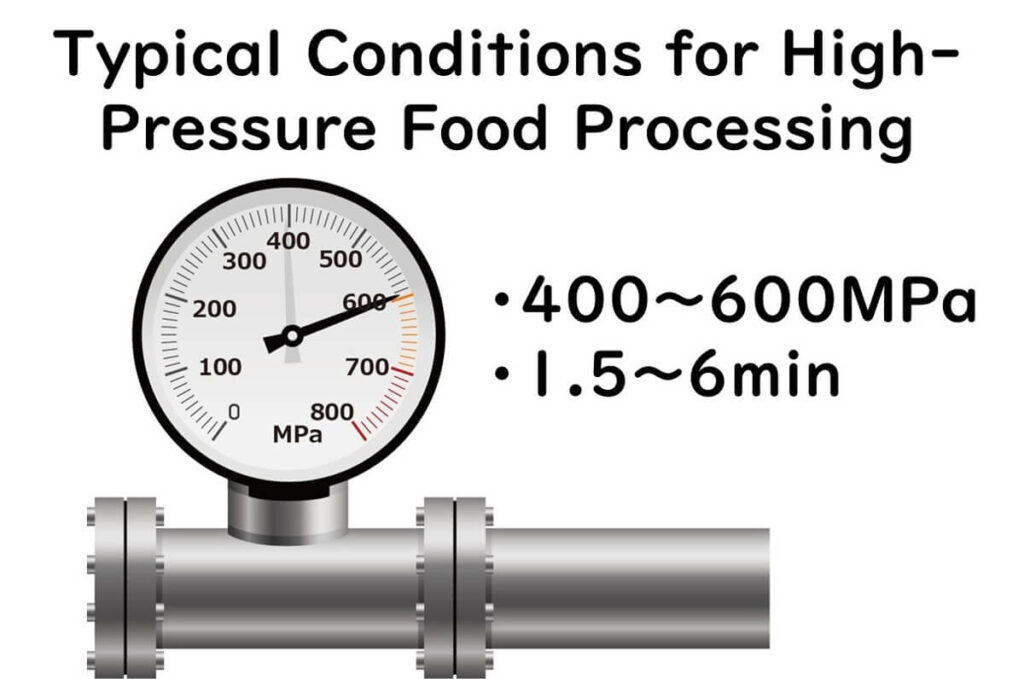
Overview of High-Pressure Processing Equipment
HPP equipment operates via two main methods:
Indirect Pressurization: A high-pressure medium is pumped into the vessel, which is more common in the food industry.
Direct Pressurization: A piston reduces the volume of the pressure vessel, increasing the pressure.
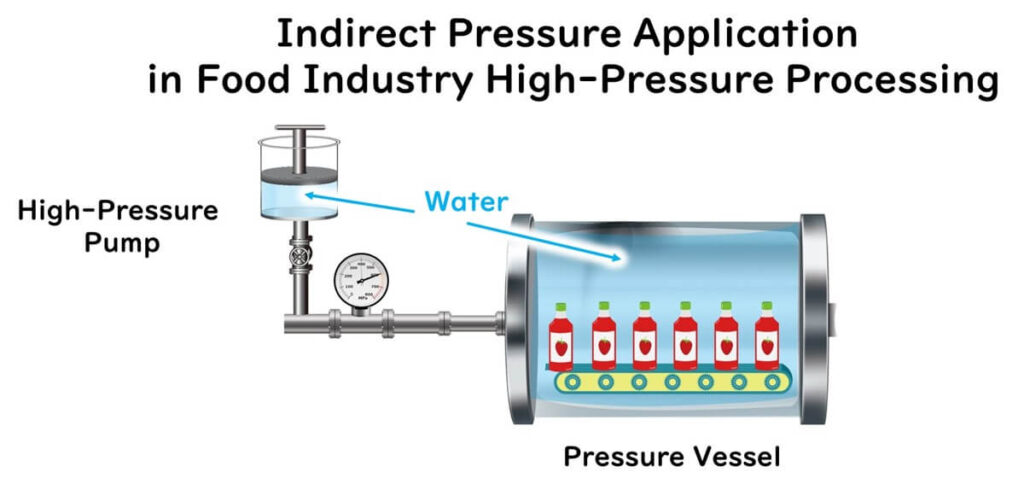
In commercial settings, pressures up to 600 MPa are standard, though laboratory systems can achieve up to 1,400 MPa. Water, as a low-compressibility fluid, is the most commonly used pressure medium. It is often cooled to 10-15°C for consistent performance.
The HPP process consists of three stages:
- Compression Stage: A pump or intensifier feeds the liquid medium into the pressure chamber, raising the pressure.
- Holding Time: The target pressure is maintained for a specific duration.
- Pressure Release Stage: The pressure is rapidly decreased.
During compression, pressure causes adiabatic heating, raising the temperature of the food, the pressure medium, and the container's interior. For instance, every 100 MPa increase results in a temperature rise of about 2-3°C. For homogenous foods such as juices, this can reach 3°C per 100 MPa at an initial temperature of 25°C. Decompression, however, may cool the food below its initial temperature if heat dissipates during the hold time.
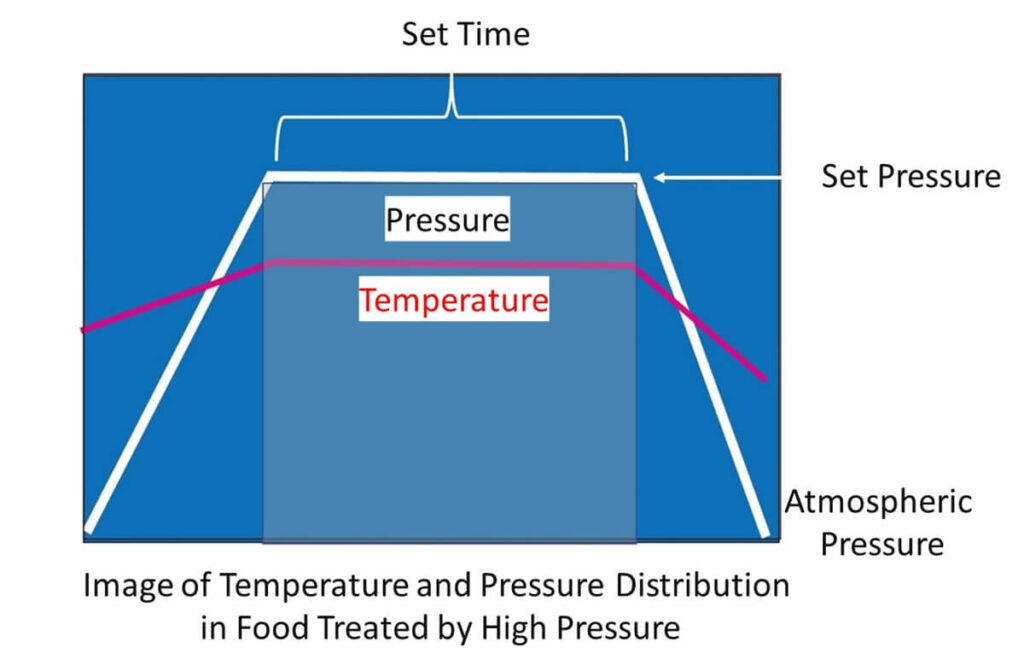
For HPP-treated foods, packaging is usually done beforehand. Vacuum-sealed packaging is preferred to eliminate air and prevent package deformation under pressure.
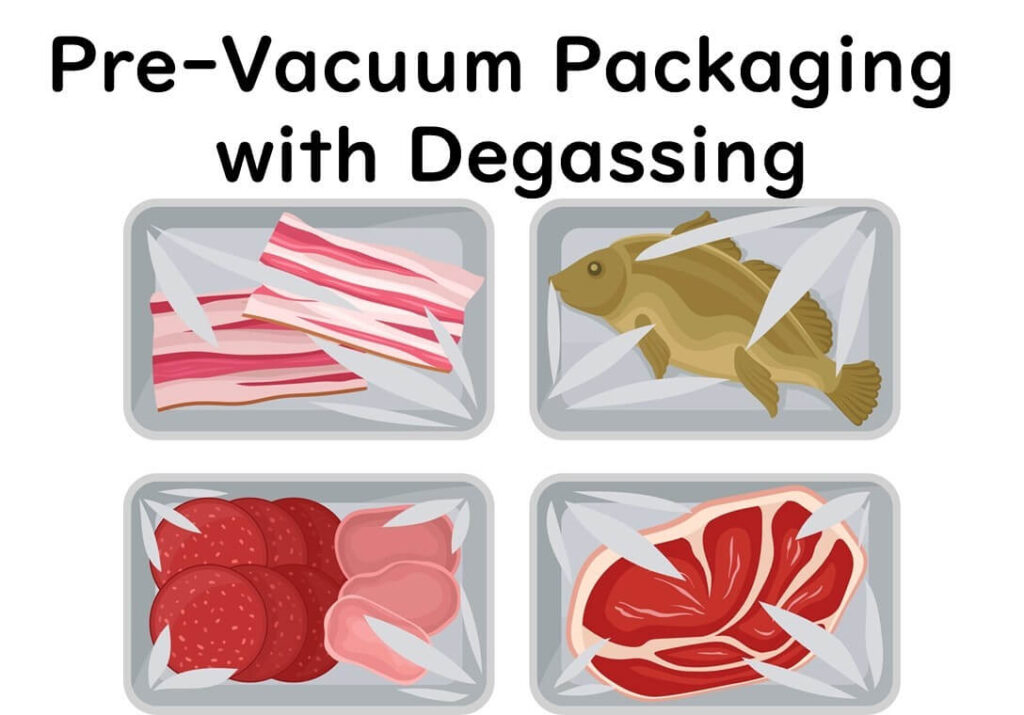
Sterilization Mechanism
High-Pressure Processing (HPP) disrupts hydrophobic and ionic bonds, leading to the partial or complete denaturation of enzymes and proteins within bacterial cells. However, it does not break covalent bonds, such as peptide bonds, preserving the food's molecular integrity.
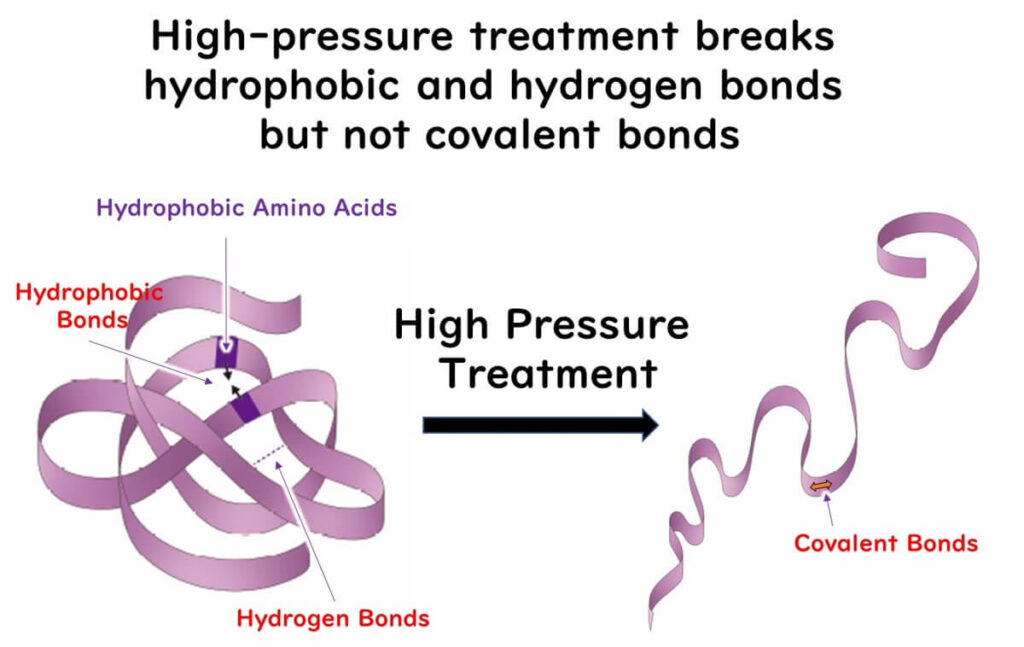
The microbial inactivation mechanism of HPP targets various cellular components, including:
- Cell Wall and Membrane: Pressure compromises the structural integrity of the cell envelope.
- Nucleic Acids and Ribosomes: Functional impairments hinder protein synthesis and replication.
- Enzymes and Proteins: Denaturation disrupts vital cellular processes.
This multi-target effect ensures that bacteria are not inactivated by a single mechanism but through a combination of impacts, with protein denaturation considered the primary mode of action under high-pressure conditions.
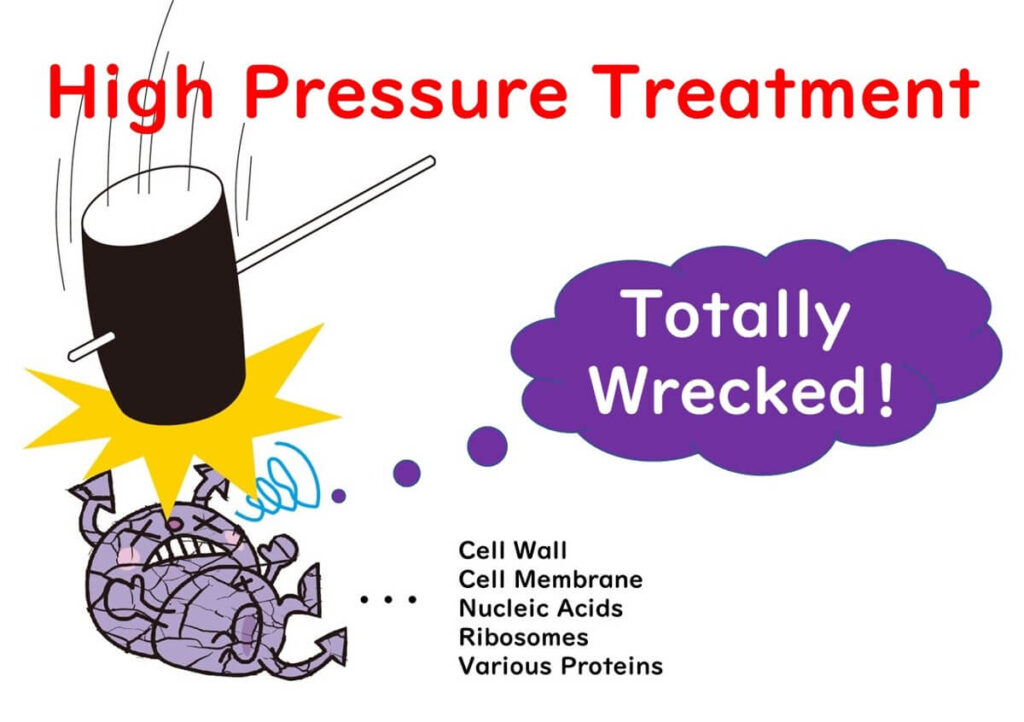
In addition to pressure, microbial inactivation is believed to result from the combined effects of other physical forces such as:
- Cavitation: Formation and collapse of vapor-filled cavities.
- Shear Stress and Turbulence: Mechanical forces disrupt cellular structures.
- Collisions and Friction-induced Heat: Generate localized increases in temperature that amplify inactivation.
This synergistic action of pressure and physical impacts makes HPP highly effective for microbial sterilization while maintaining food quality.
Factors Influencing the Effectiveness of High-Pressure Processing
Magnitude of Pressure
Numerous studies on High-Pressure Processing (HPP) have demonstrated that microbial inactivation increases proportionally with both the applied pressure level and exposure time.
For instance, Lavinas et al. reported that the D-value (the time required to reduce the microbial population by one logarithmic cycle) for Escherichia coli in apple juice (initial population approximately 10610^6106 CFU/mL) was as follows:
- 250 MPa: 16.4 minutes
- 300 MPa: 11.3 minutes
- 350 MPa: 2.4 minutes
- 400 MPa: 1.2 minutes
This data highlights the dramatic reduction in D-value as pressure increases, making HPP highly effective for microbial control.
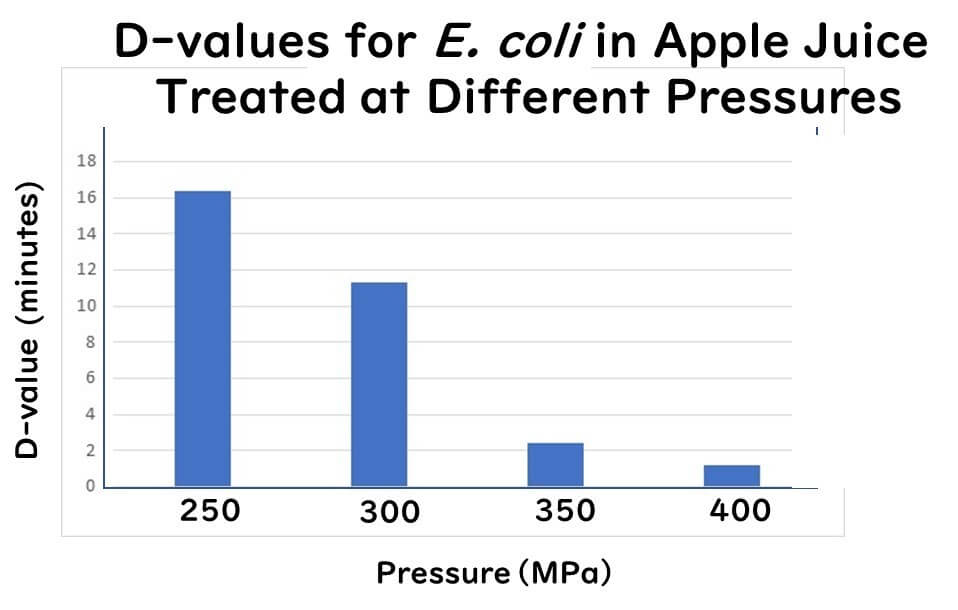
Compiled from data in Lavinas et al.(2008)
Processing Time
Similarly, increasing the duration of high-pressure processing at a constant pressure also enhances microbial inactivation. According to Lavinas et al.(2008), the logarithmic reduction of E. coli in apple juice processed at 400 MPa was as follows:
- 0.5 minutes: 2.19 log reduction
- 1.0 minute: 2.95 log reduction
- 1.5 minutes: 4.2 log reduction
This data indicates that both pressure and processing time are critical factors in achieving effective microbial sterilization.
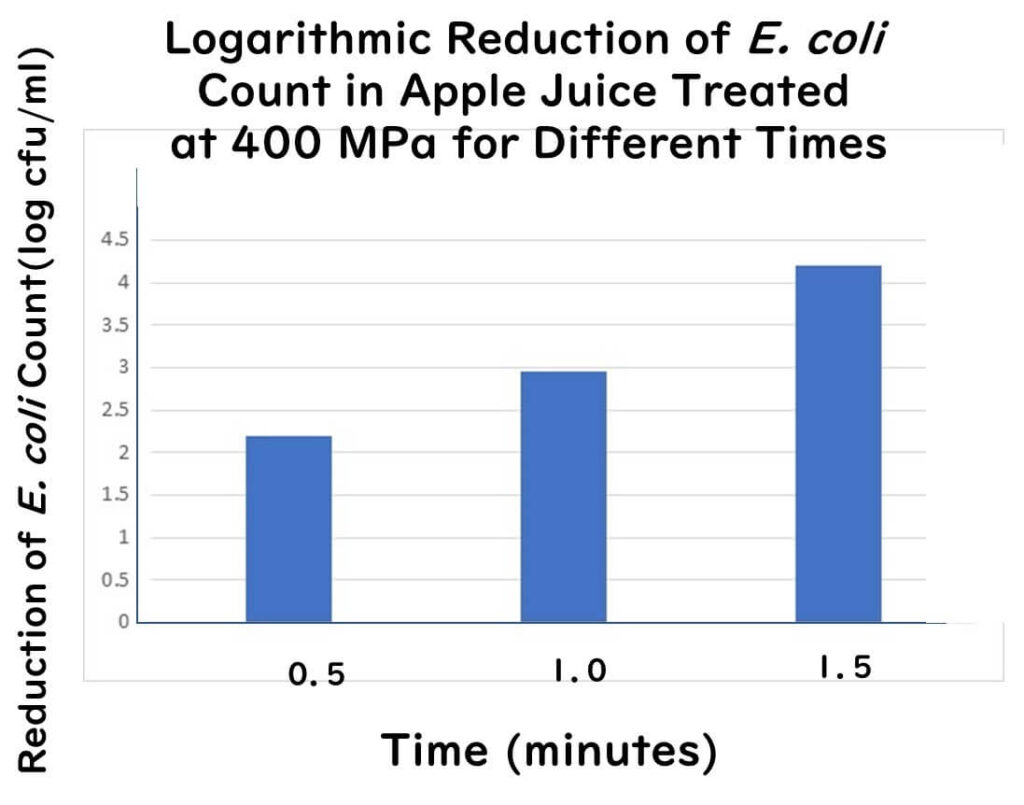
Compiled from data in Lavinas et al.(2008)
Temperature and Depressurization Rate During High-Pressure Processing
Various studies have explored the effects of temperature and depressurization rate during high-pressure processing, but the results often present contrasting conclusions. Currently, the relationship between temperature or depressurization rate and sterilization efficiency in high-pressure processing remains inconclusive.
For further detailed discussions, see:
Factors Affecting Microbial Inactivation during High Pressure Processing in Juices and Beverages: A ReviewJ Food Prot (2020) 83 (9): 1561–1575.
Water Activity of the Target Food
Water activity (aw) plays a crucial role in the inactivation of bacteria and yeast during high-pressure processing.
Generally, as water activity decreases, microbial growth is inhibited; however, microbes may also gain resistance to environmental stresses such as heat and pressure. In high-pressure processing, low water activity reduces the sterilizing effect. Although the exact mechanism remains unclear, it is speculated that proteins stabilize under conditions of reduced water activity.
Example: High-Pressure Processing of E. coli
The diagram below illustrates the relationship between water activity and high-pressure processing of Escherichia coli at 25°C. For instance:
At 450 MPa and a water activity of 0.992, the microbial reduction exceeds 5 logs.
At 450 MPa and a water activity of 0.850, the microbial reduction is approximately 3.5 log.
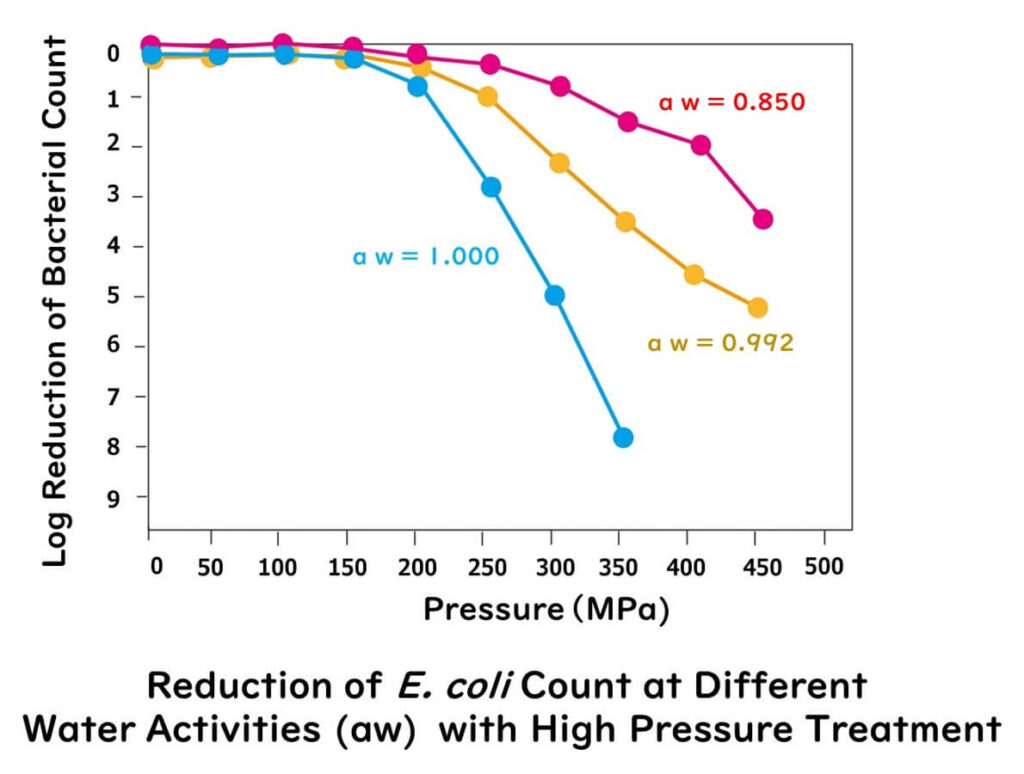
The figure above was drawn from data on the relationship between water activity and high-pressure treatment from the following paper .
Synergistic and Antagonistic Effects of Combined Subzero Temperature and High Pressure on Inactivation of Escherichia coli
Appl.Enbiron.Microbiol.72.150-156(2006)
Why Does Low Water Activity Reduce the Effectiveness of High-Pressure Processing?
This phenomenon has not yet been fully elucidated, but the following hypothesis has been proposed:
- Hydration Waters and Protein Flexibility:
- In high water activity environments, water molecules surrounding proteins maintain their structural flexibility.
- High-pressure processing exposes hydrophobic sites and alters the active sites of enzymes, contributing to microbial inactivation.
- Low Water Activity Effects:
- Under low water activity conditions, hydration waters are reduced, and protein structures become rigid.
- This rigidity prevents the exposure of hydrophobic sites during high-pressure processing, making enzymes and microbial cells more resistant to inactivation.
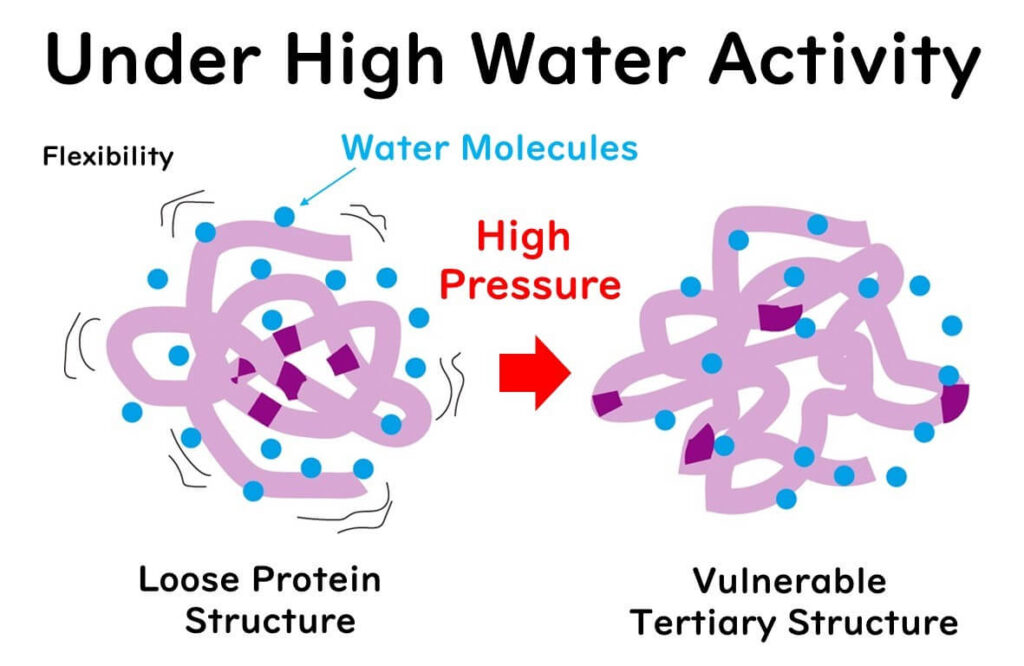
As a result, high-pressure processing may be less effective for foods with low water activity. Practical applications of this technology to dry or low aw foods may therefore be limited, as the microbial and enzymatic resistance to pressure diminishes its sterilizing potential.
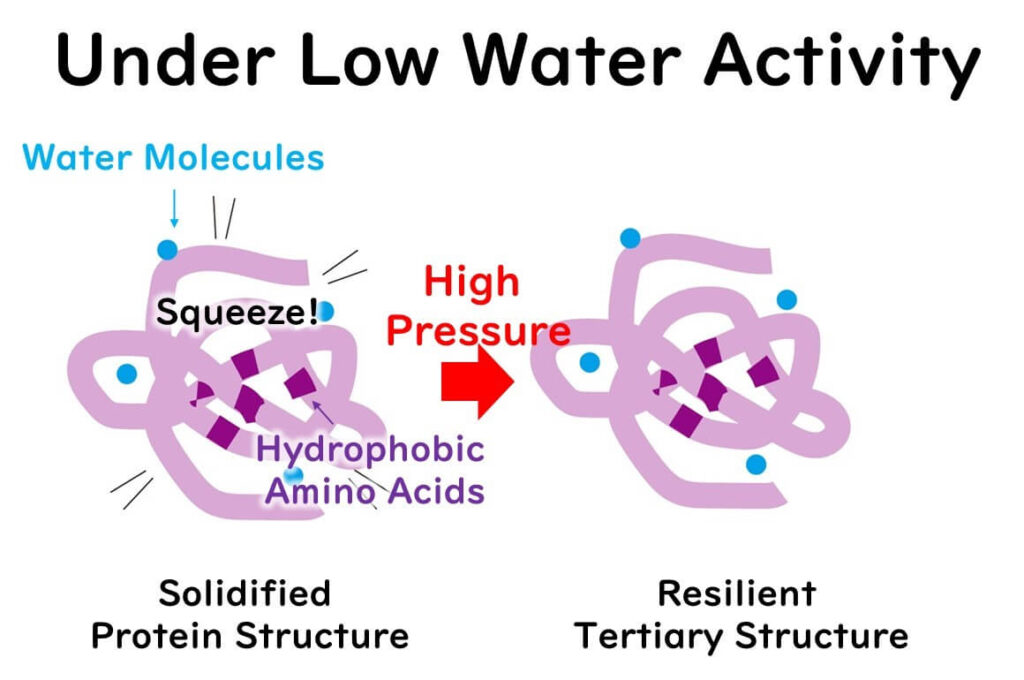
The pH of the Target Food
The pH of the target food significantly influences the inactivation of microorganisms during high-pressure processing. It has been consistently observed that lower pH levels increase the susceptibility of bacterial vegetative cells, spores, yeast, and molds to high-pressure treatment.
Example: Effect of pH on High-Pressure Processing of E. coli O157
A study investigated the effect of high-pressure treatment on the survival of pressure-resistant Escherichia coli O157in orange juice with varying pH levels ranging from 3.4 to 5.0.
Experimental Setup:
- Sample: Commercially sterile orange juice
- pH Levels: Adjusted to 3.4, 3.6, 3.9, 4.5, or 5.0
- Inoculation: E. coli O157at 10⁸ CFU/mL
- Treatment Conditions: 400 MPa at 20°C
Results:
The diagram below demonstrates that the lower the pH of the orange juice, the greater the sterilizing effect of high-pressure processing.
Why Does Low pH Enhance the Effectiveness of High-Pressure Processing?
A lower pH is believed to enhance the sterilizing effect of high-pressure processing due to the inability of surviving cells to quickly repair damage under acidic conditions. The stress caused by the combination of high pressure and low pH impairs the cells' recovery mechanisms, leading to greater inactivation.
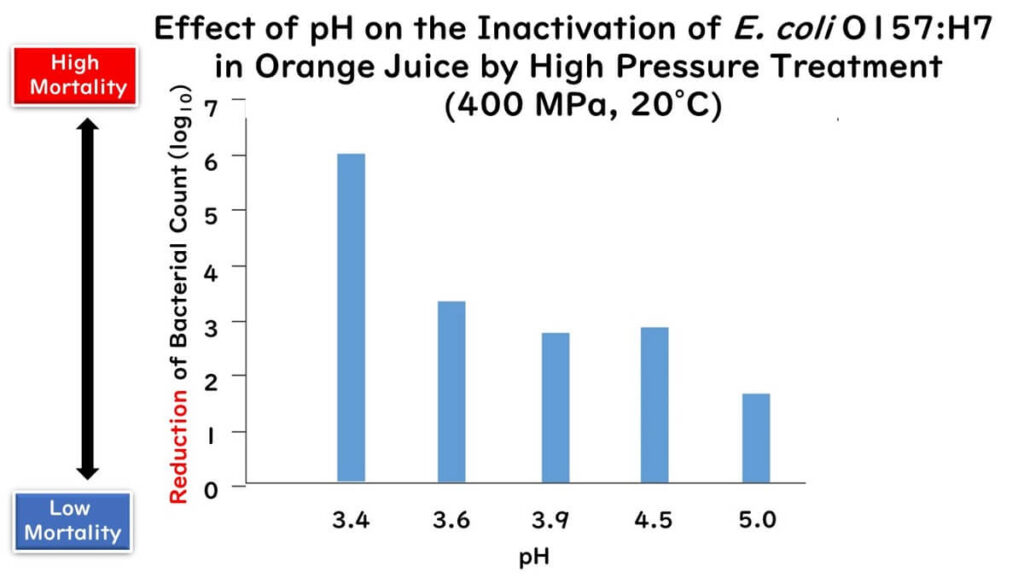
The diagram above was created using data extracted from the following study:
Inactivation of Escherichia coli O157:H7 in orange juice using a combination of high pressure and mild heat. J Food Prot, 62(3):277-9(1999)
Application Examples of Microbial Sterilization
High-pressure processing (HPP) has been widely applied to pre-packaged products, including juices, sauces, dips, stir-fries, meat products, and ready-to-eat (RTE) foods. In the European Union, there is growing interest in HPP as an alternative to pasteurization for milk.
Over the past 30 years, numerous studies have investigated the application of HPP for various food products. Below are three specific examples that illustrate its effectiveness.
Application Example 1: Sterilization of Orange Juice
Juices are highly susceptible to microbial spoilage and enzymatic activity, which limit their shelf life. High hydrostatic pressure (HHP) is effective in inactivating both microbes and enzymes.
Study Overview:
A study evaluated the effects of mild heating combined with high hydrostatic pressure on Staphylococcus aureus, Escherichia coli O157, and Salmonella in apple, orange, apricot, and sour cherry juices.
Experimental Conditions:
- Pressure Range: 250–450 MPa
- Temperature Range: 25–50°C
- Processing Time: 0–60 minutes (depending on the desired inactivation level)
Key Findings:
- At 250 MPa and 30°C, a 20-minute treatment achieved a 5-log reduction of S. aureus, E. coli O157, and Salmonella.
- A 5-minute treatment at 350 MPa and 40°C resulted in complete microbial inactivation.
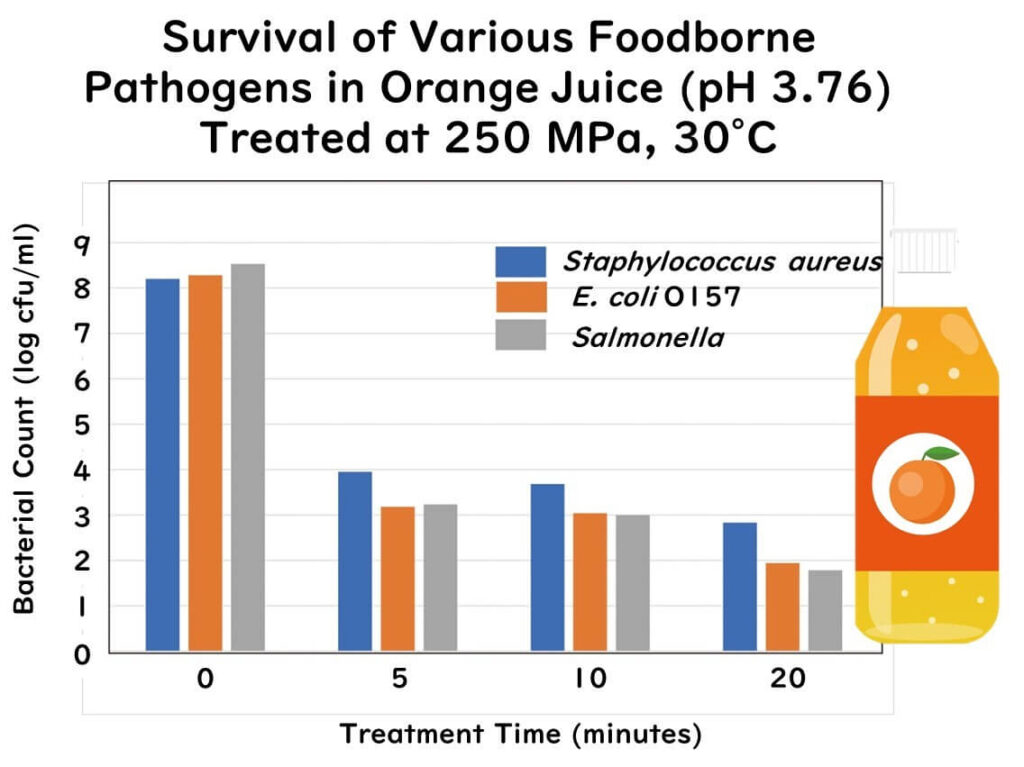
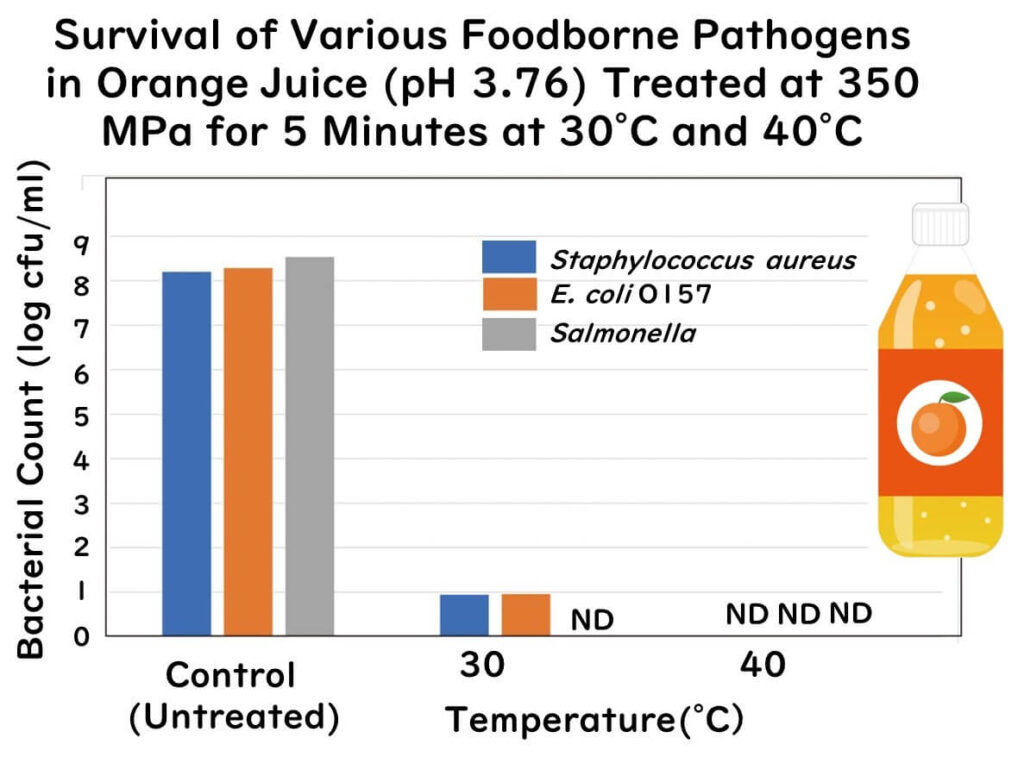
The above two diagrams were created using data from the following study.
Efficiency of high pressure treatment on inactivation of pathogenic microorganisms and enzymes in apple, orange, apricot and sour cherry juices
Food Control 17(1):52-58(2006)
Application Example 2: Sterilization of Chicken Meat
The application of high hydrostatic pressures ranging from 300 MPa to 600 MPa was studied for its effects on microbial populations, meat quality, and sensory properties in chicken breast meat.
Key Findings:
- Microbial Safety:
- 450 MPa and 600 MPa nearly eliminated harmful bacteria (Salmonella, E. coli O157, and Listeria), significantly improving safety.
- Microbial reduction:
- 450 MPa: 4–8 log (CFU/g) reduction over 3–14 days.
- 600 MPa: 6–8 log (CFU/g) reduction over 7–14 days.
- Quality and Sensory Effects:
- 300 MPa reduced flavor intensity, aroma, and juiciness.
- 450 MPa caused the weakest aroma, but increased L, a, b* color values, cooking loss, and color intensity.
- Firmness and chewiness increased with higher pressure.
- Lipid Oxidation:
- Pressures above 450 MPa induced lipid oxidation, which may negatively impact shelf life.
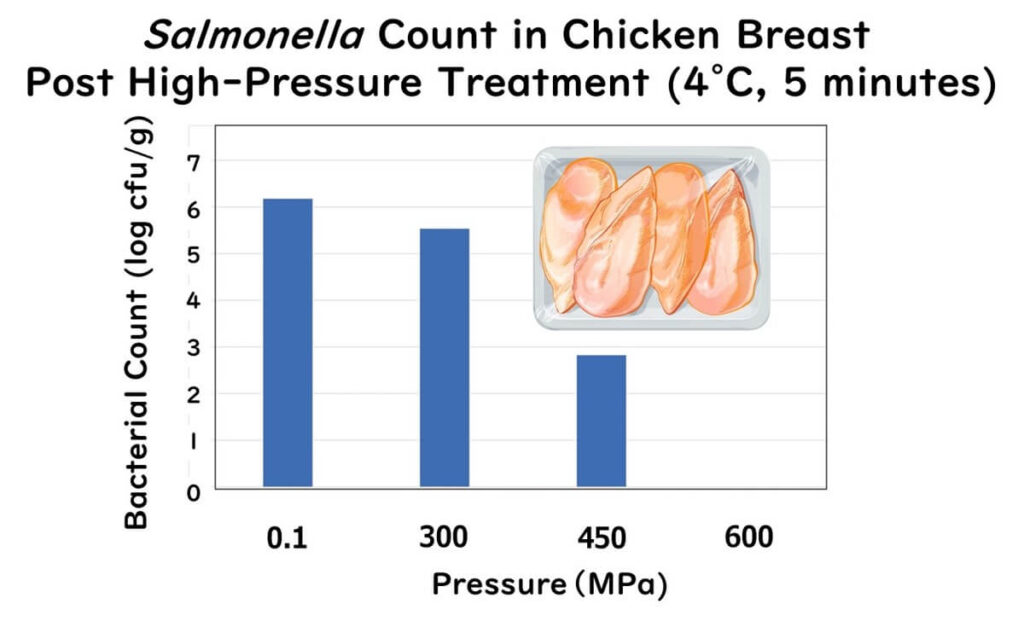
Conclusion:
HPP effectively reduces bacterial spoilage and extends shelf life for chicken breast fillets. However, pressures above 450 MPa can adversely affect sensory and quality properties, necessitating further studies to balance microbial safety and product quality.
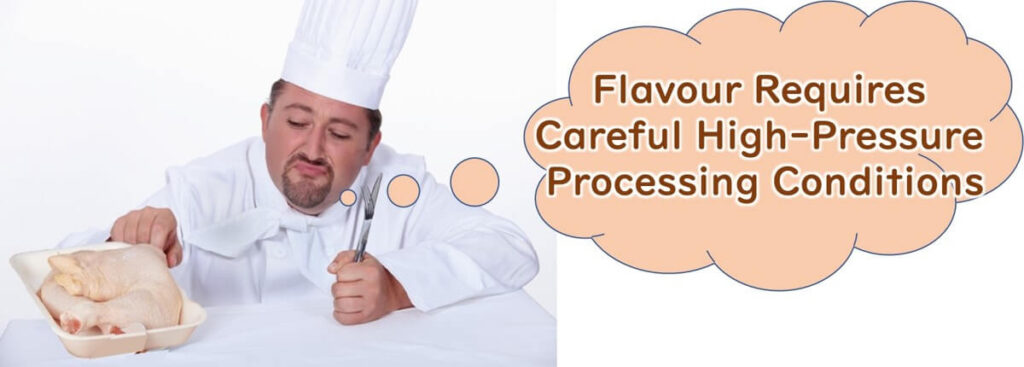
The effect of high pressure on microbial population, meat quality and sensory characteristics of chicken breast fillet
Food Control, 22, 6-12(2011)
Application Example 3: Sterilization of Liquid Eggs with a High-Pressure Homogenizer
The demand for liquid eggs has significantly increased in both commercial and domestic settings due to their convenience and versatility. However, ensuring the safety of liquid egg products requires eliminating Salmonella. While low-temperature pasteurization is commonly used, it can negatively impact key functional properties of egg whites, such as:
- Coagulation capacity
- Foaming ability during whipping
- Emulsion formation
- Adhesion bonding
- Digestibility
To address these issues, high-pressure homogenization (HPH) has emerged as a promising alternative technology.
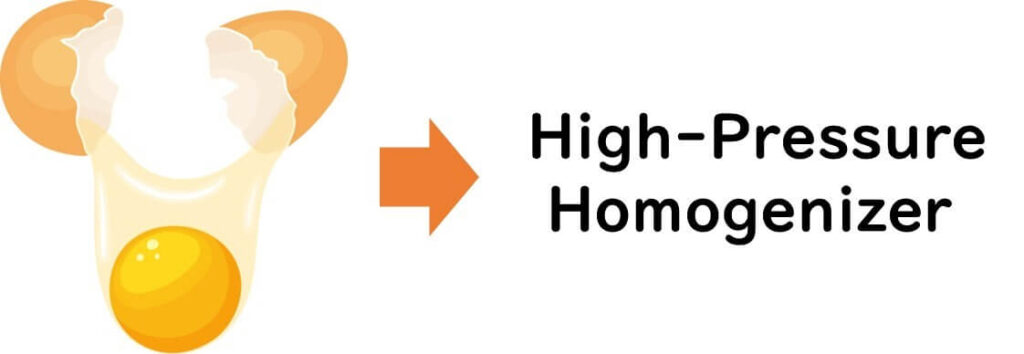
High-Pressure Homogenization Overview
High-pressure homogenizers (HPH) are specifically designed for the continuous processing of fluid foods. These devices push fluids through a narrow gap in the homogenizer valve, causing rapid acceleration and inducing physical phenomena such as:
- Cavitation
- Shear stress
- Turbulence
These combined forces effectively disrupt microbial cells, achieving sterilization while preserving the functional qualities of the liquid food.
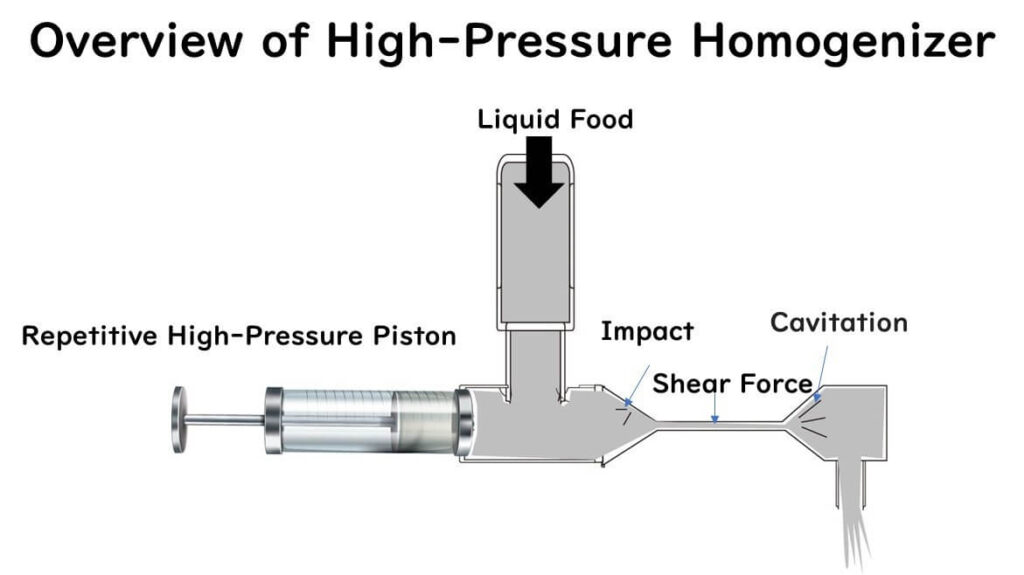
Effect of high pressure homogenisation on microbial inactivation, protein structure and functionality of egg white.
Food Research International, 62, 718-725(2014)
Foods Unsuitable for High-Pressure ProcessingWhile high-pressure processing (HPP) is versatile and can be applied to a wide range of foods, certain types are not well-suited for this technology due to their physical characteristics or structural limitations.
Examples of Foods Unsuitable for HPP:
While high-pressure processing (HPP) is versatile and can be applied to a wide range of foods, certain types are not well-suited for this technology due to their physical characteristics or structural limitations.
Examples of Foods Unsuitable for HPP:
- Aerated Foods:
- Foods containing air bubbles, such as bread, cakes, or other baked goods, may suffer from structural damage during HPP. The pressure compresses and disrupts their porous structures, leading to undesirable changes in texture and appearance.
- Whole or Fresh-Cut Fruits and Vegetables:
- These items may experience cell wall damage under high pressure, resulting in textural degradation or increased susceptibility to spoilage.
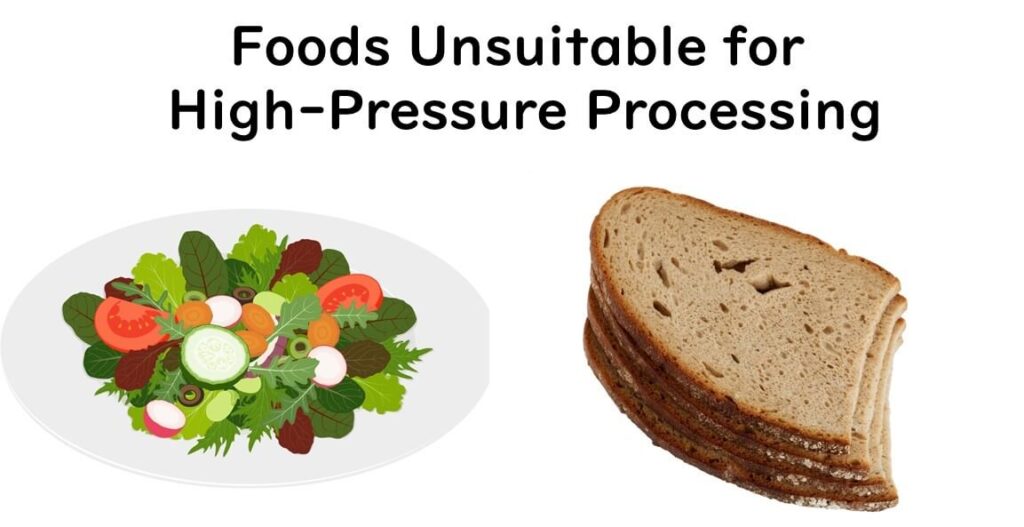
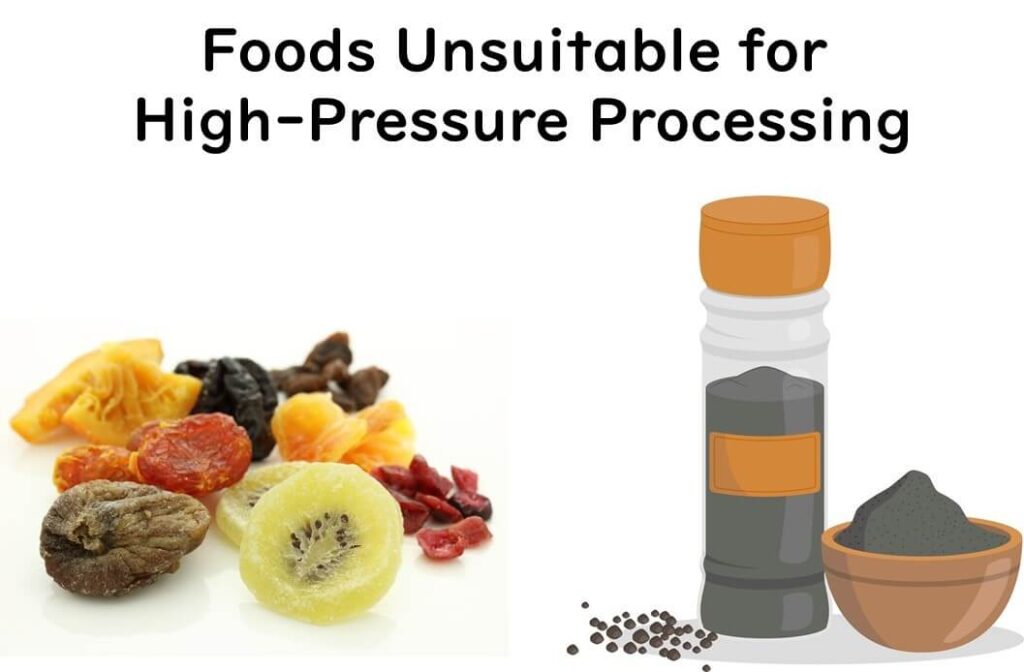
Microorganisms Unsuitable for High-Pressure Processing
HPP is highly effective at inactivating vegetative cells of bacteria, yeasts, and molds, but it is not effective against bacterial spores in its standard form.
Spores and High-Pressure Processing:
Combination Treatments: Inactivation of spores may be achieved when HPP is combined with other methods, such as mild heat treatment (pressure-assisted thermal sterilization, or PATS).
Resistance to Pressure Alone: Bacterial spores are highly resilient and can survive standard HPP conditions.
Applications of High-Pressure Processing Beyond Microbial Sterilization
High-pressure processing (HPP) is not limited to microbial sterilization. It is also used in various food technology applications and the development of innovative products. These include:
- Freezing
- Enzyme Control
- Low-Temperature Gelatinization of Starch
- Protein Unfolding
- Shell Peeling of Shellfish
- Enhancement of Mass Transfer Phenomena
As this blog focuses on food microbiology, these applications will not be elaborated further here.
The History of High-Pressure Processing Research in Global Food Studies
Over the past 30 years, significant advancements have been made in both research and industrial applications of high-pressure processing (HPP).
Historical Milestones in HPP Research:
- Early Discoveries (Dr. Bridgman):
- The foundation of HPP research was laid by Dr. Percy W. Bridgman, Nobel Prize winner in Physics (1946). He discovered that applying 500–600 MPa hydrostatic pressure caused coagulation of egg yolk and egg white within shell-encased eggs.
- Advancements in Japan (Dr. Rikimaru Hayashi):
- Practical food applications of HPP began in Japan in 1987, when Dr. Rikimaru Hayashi of Kyoto University proposed applying high-pressure technology to food processing.
- In 1990, Japan commercialized its first high-pressure processed products, including juices, jellies, and jams.
- HPP applications soon expanded to other foods such as meats, fish, rice pudding, beef ham, and sake.
- Global Expansion:
- Following Japan's pioneering efforts, the technology was adopted internationally, leading to widespread research and development.
- EFSA’s Assessment (2022):
- On March 8, 2022, the European Food Safety Authority (EFSA) released an assessment report on the safety and efficacy of high-pressure processed foods. This report is expected to further accelerate the global adoption of HPP technology.
For detailed insights, see:
"The efficacy and safety of high-pressure processing of food"
(European Food Safety Authority, 2022).
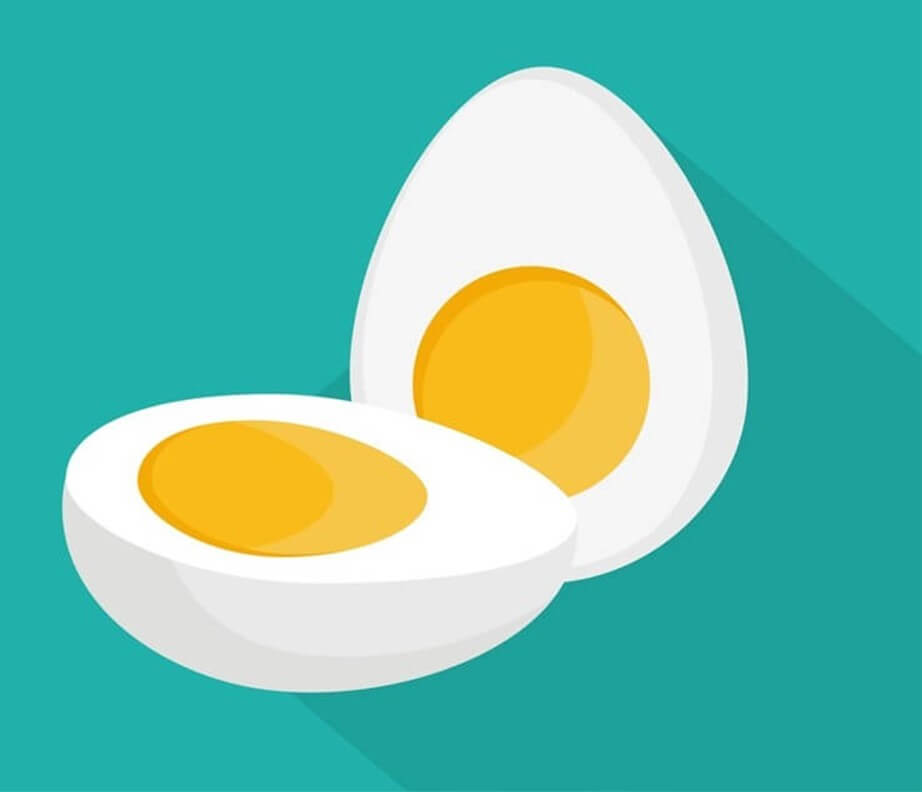