In our ongoing exploration of food preservatives, we delve into the fascinating world of nisin, a natural antimicrobial agent that has carved a niche for itself in the global food industry. Originally discovered in fermented milk in 1928, nisin has been celebrated for its effectiveness and safety, receiving approval from various health authorities worldwide, including the FAO/WHO and the U.S. FDA. This article examines nisin’s journey from a humble natural discovery to a cornerstone in food preservation, highlighting its unique properties, mechanisms of action, and its emerging significance in the medical field against antibiotic-resistant bacteria. Join us as we uncover how nisin, a polycationic peptide and a member of the bacteriocins family, continues to make significant contributions to both food safety and human health, proving itself as a vital tool in our antimicrobial arsenal.
What is Nisin?
Nisin was first discovered in 1928 from fermented milk. The United Kingdom marked its first commercial antimicrobial debut in 1953. By 1969, it was recognized as a safe food additive by the FAO/WHO. In 1988, the U.S. FDA approved its use in processed cheeses, and in 2006, its safety was reaffirmed by the EFSA Panel on Food Additives, Flavourings, Processing Aids, and Materials in Contact with Food in the EU.
Today, nisin is widely used in over 50 countries as a natural antimicrobial agent. The U.S. Food and Drug Administration (FDA) classifies nisin as GRAS (Generally Recognised as Safe).
Like the shirako and polylysine discussed in the previous article, nisin is a low molecular weight polypeptide made up of about 30 amino acids, which contributes to its thermal stability. This feature makes it an excellent choice for a food additive.
Furthermore, as previously mentioned, polycationic antimicrobial peptides like nisin and polylysine play a crucial role in the biological defense systems of all organisms, ranging from bacteria to invertebrates and mammals. In animals, antimicrobial peptides are known to be produced by epithelial cells of the urinary and digestive systems and lymphocytes in response to bacterial polysaccharides. For instance, in humans, Paneth cells in the intestines secrete antimicrobial peptides to prevent pathogenic microbes from causing inflammation.
Plants also produce antimicrobial peptides during various stages of growth, including in leaves, roots, flowers, seeds, and stems. This antimicrobial function is known to play a role in the immunity of amphibians as well. Even invertebrates such as spiders, clams, and shrimp use antimicrobial peptides to prevent bacterial infections.
Thus, antimicrobial peptides can be seen as a natural antimicrobial system produced when organisms are injured or infected, with nisin being one such peptide.
Reference: The significance of antimicrobial peptides, including nisin, was spotlighted in the 1990s for their antimicrobial properties, leading to the consolidation of basic information into a database. As a result, in 2003, the antimicrobial peptide database (APD) was established online by the University of Nebraska. This site features over 2600 antimicrobial peptides from almost all biological sources, including animals, plants, bacteria, protozoa, and archaea. Anyone interested should certainly take a peek at this fascinating database.
The Structure of Nisin
Nisin, as introduced in our previous article, is a cationic antimicrobial peptide, similar to shirako and polylysine. It is composed of 34 amino acids, making it a cationic peptide.
There are various forms of nisin, among which nisin A is the most extensively studied. Nisin A was first discovered in Lactococcus lactis, commonly isolated from dairy products. A close variant, nisin Z, also derived from Lactococcus lactis, differs from nisin A in that the 27th amino acid residue is asparagine instead of histidine. Both are excellent antimicrobials, though nisin Z is reputed to have better diffusion rates and solubility under neutral pH conditions.
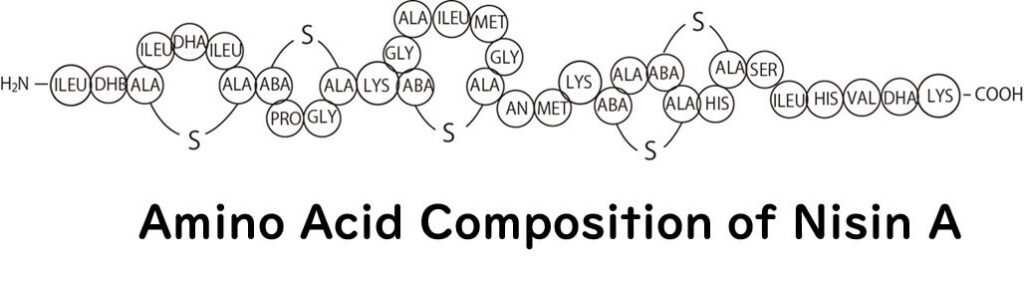
Generally, antimicrobial peptides, including nisin and previously discussed polylysine, consist of 10 to 50 amino acids. They often contain a higher proportion of cationic amino acids like lysine, arginine, and histidine, giving the peptide a net positive charge, or cationic nature.
Nisin belongs to a group of antimicrobial peptides known as lantibiotics. A distinctive feature of this group is the inclusion of unusual amino acids in the peptide structure. Specifically, they contain hydrophobic amino acids like serine and threonine, as well as five lanthionine rings formed through thioether cross-linking.
These properties — the charged cationic nature and the inclusion of hydrophobic amino acids — impart amphipathic characteristics to the peptide. This amphipathicity significantly contributes to nisin's antimicrobial mechanism, which we'll explore further on.
The Antimicrobial Mechanism of Nisin
The exact mechanism by which antimicrobial peptides like nisin inhibit microbial growth is not yet fully understood. The most widely accepted action mechanism for nisin involves its interaction with microbial cell membranes, creating pores in them. Recent studies also propose mechanisms such as inhibition of nucleic acid and protein synthesis.
However, as mentioned in another article, the primary target for antimicrobial agents that inhibit microbial growth is the cell membrane.
Basic Aspects of the Germicidal Mechanism: For a fundamental understanding of antimicrobial mechanisms, please refer to the article
Essential Common Understanding of Disinfectant Mechanisms in Food Factories
- First Stage:
Regardless of the specific mechanism, the initial stage involves the attraction between the peptide and the target cell. The key factor here is the attraction between the positively charged basic amino acid residues of the peptide and the negatively charged surfaces of microbial cells.
For basics on microbial cell surface charges, refer to the article
The Food Factory - Quaternary Ammonium Salts (Benzalkonium Chloride)
In contrast, these positively charged cationic peptides do not electrostatically attract to the cell membranes of eukaryotic hosts. This is because, unlike bacterial membranes, eukaryotic cell membranes are not negatively charged. This distinction is also explained in another article.
For basics on the difference in charges between bacterial and eukaryotic cell membranes, see the relevant section in
From Food Preservation to Antibiotic Alternatives: The Antimicrobial Power of Polylysine and Protamine
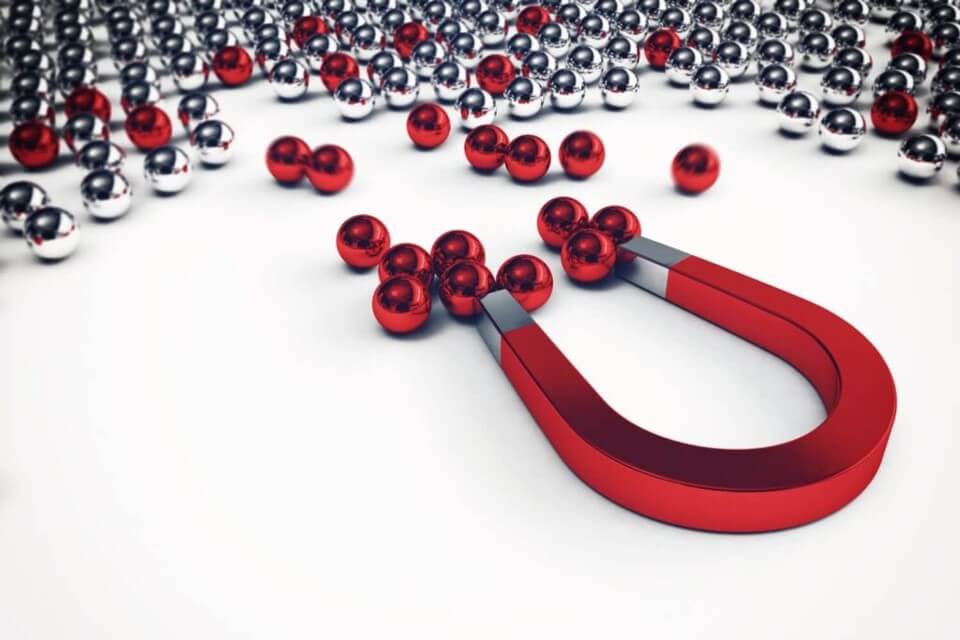
- Second Stage:
The second step involves the interaction of the peptide's hydrophobic amino residues with the hydrophobic parts of the microbial cell membrane, disrupting it.
The mechanism by which nisin disrupts cell membranes has not reached a full consensus. The common view is that at low peptide concentrations, the peptide lies horizontally across the lipid bilayer. However, as peptide concentration increases, two models have been proposed:
Transmembrane Pore Model: At higher concentrations, the peptides orient vertically rather than horizontally, piercing through the lipid bilayer, creating pores.
Carpet Model: As peptide concentration increases, peptides lay across the lipid bilayer like a carpet. This results in the formation of micelles and ultimately pores in the cell membrane.
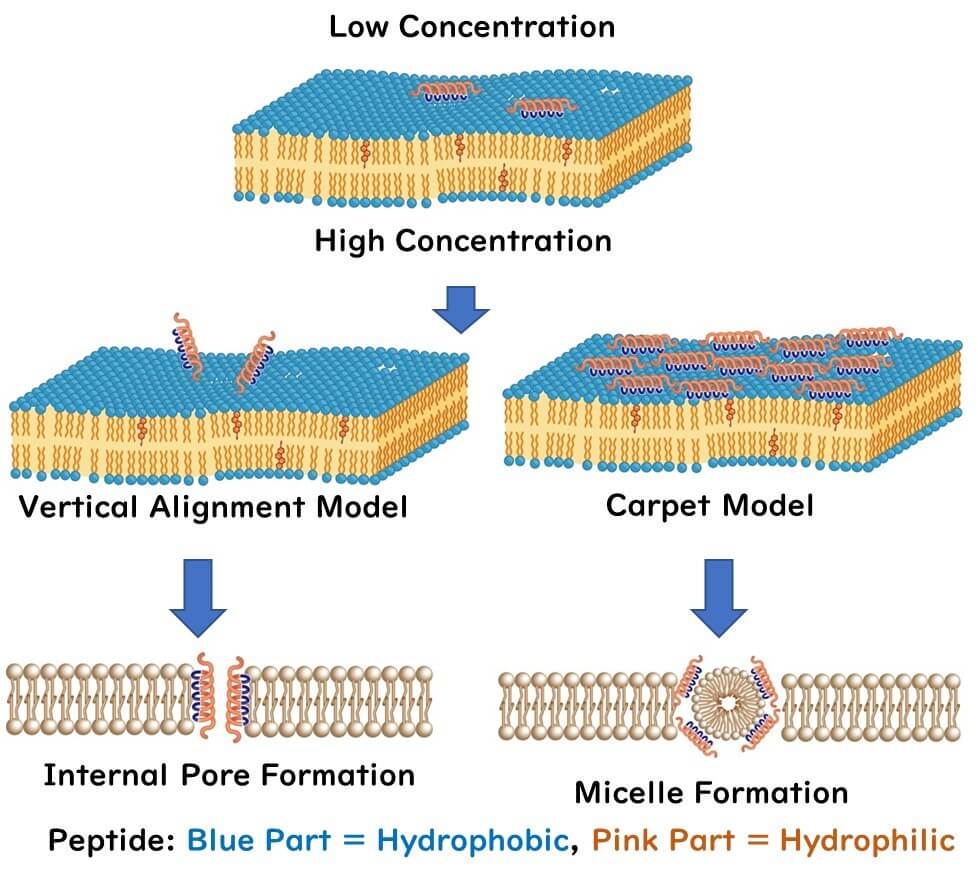
Regardless, these models have been demonstrated using in vitro experiments with artificial cell membranes, not actual cells. Therefore, it's yet to be conclusively determined if these mechanisms also occur in real microbial cells.
In any case, it's sufficient to generally understand nisin's antimicrobial mechanism as described above, involving adsorption to and disruption of the cell membrane.
The Influence of pH on Nisin's Antimicrobial Effectiveness
Research indicates that nisin's antimicrobial activity increases as the pH decreases. This phenomenon has been observed with other foodborne pathogens as well. For example, a study examining the antimicrobial effects on Bacillus cereus found that nisin shows stronger antimicrobial activity at pH 6.30 and 5.75 at 30°C, compared to a pH of 7.0. Similarly, studies on Listeria monocytogenes and Staphylococcus aureus have reported that a reduction in pH (from 7.92 to 5) enhances nisin's antimicrobial effectiveness.
The reason why nisin's antimicrobial activity generally increases in mildly acidic conditions rather than neutral ones remains unclear, though many hypothesize that it is due to nisin's higher solubility and stability at lower pH values.
Here's the blog author's reasoning on this mechanism:
The reason nisin shows higher antimicrobial effectiveness in mildly acidic conditions could be due to its increased positive charge in such environments. Nisin's peptide chain is composed of amino acids, each possessing an amino group (-NH2) and a carboxyl group (-COOH). Following the principle of the isoelectric point of amino acids, in acidic conditions where there is an excess of H+ ions, the amino groups tend to gain a positive charge through the reaction NH2 + H+ → NH3+, while the carboxyl groups remain as -COOH (they do not dissociate to COO+ + H+). Therefore, in an acidic environment, nisin is more likely to carry an overall positive charge.
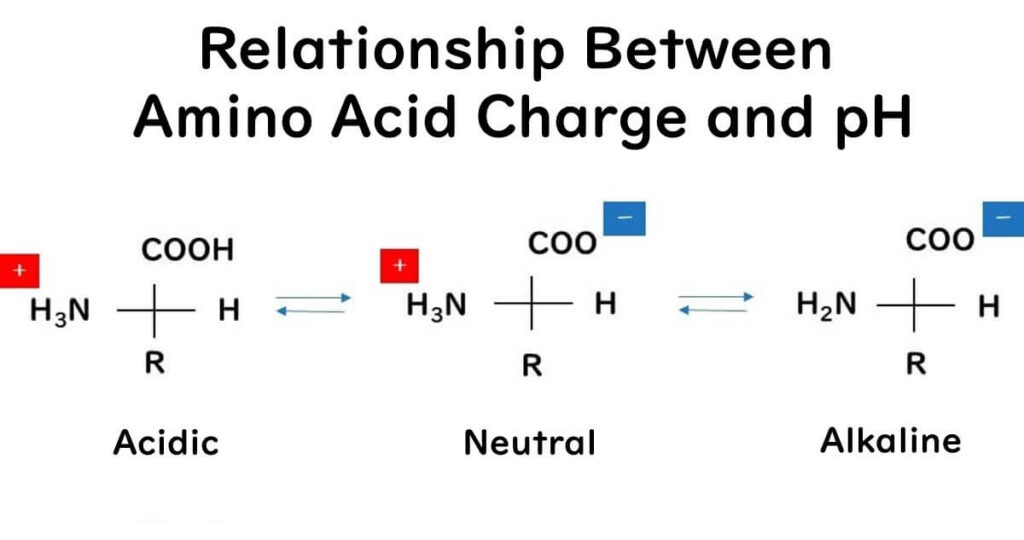
This increase in positive charge is significant because, as mentioned earlier, bacterial surfaces are negatively charged. The attraction between the positive and negative charges makes it easier for nisin to penetrate bacterial cells, enhancing its antimicrobial action.
Global Food Industry Practices Involving Nisin
As previously mentioned, nisin is now used in over 50 countries as a natural antimicrobial agent in various food products. The United States Food and Drug Administration (FDA) classifies nisin as GRAS (Generally Recognised as Safe). It is particularly expanded in its use as a natural antimicrobial in dairy products, juices, meats, and vegetables.
Recently, there has been a surge in research aiming to enhance the antimicrobial properties of substances using nanotechnology. By encapsulating antimicrobial agents in nanoparticles, nisin can be distributed with an increased surface area, improving its controlled release properties.
Moreover, in the realm of food packaging, there's a growing trend in the development of active packaging, which incorporates antimicrobial substances directly into the packaging materials. During the incorporation process, the heat resistance of the antimicrobial agents becomes crucial due to the thermal processing involved. As previously discussed, antimicrobial peptides like nisin and polylysine, introduced in earlier articles, are less susceptible to denaturation by heat compared to proteins. This makes antimicrobial peptides suitable for integration into polymer resins, thus they are seen as promising for applications in antimicrobial films.
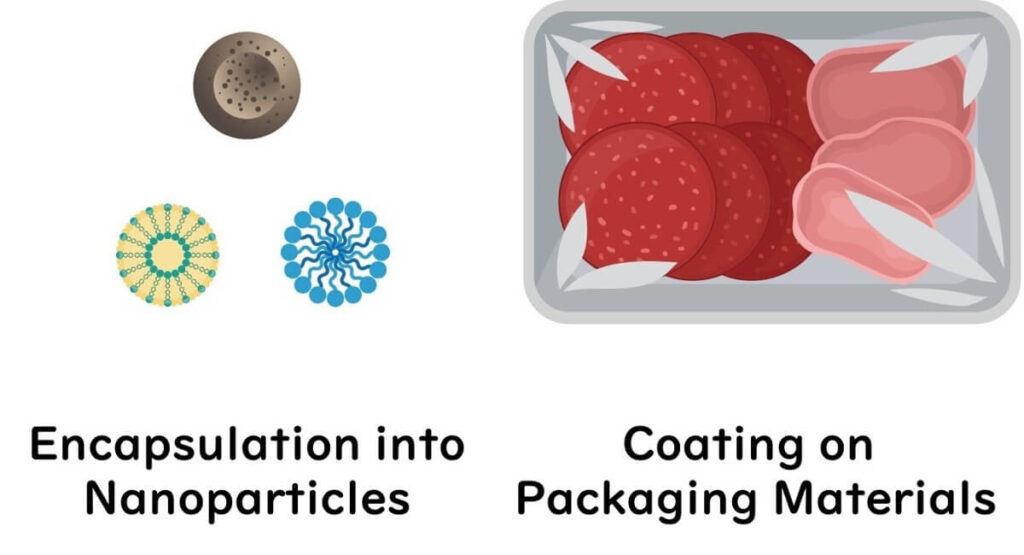
Nisin in Medical Fields: A Response to Antimicrobial Resistance
As previously discussed, while nisin has been widely used for food preservation for decades, it has garnered significant attention in the medical field since the 2000s. This interest is driven by the urgent issue of rapidly emerging drug-resistant bacteria.
Methicillin-resistant Staphylococcus aureus (MRSA) and Vancomycin-resistant Enterococci (VRE) pose serious healthcare challenges globally, often being the culprits behind nosocomial infections and urinary tract infections. For instance, MRSA infections account for about 70% of all Staphylococcus infections in intensive care units, leading to severe complications like sepsis and post-surgical pneumonia.
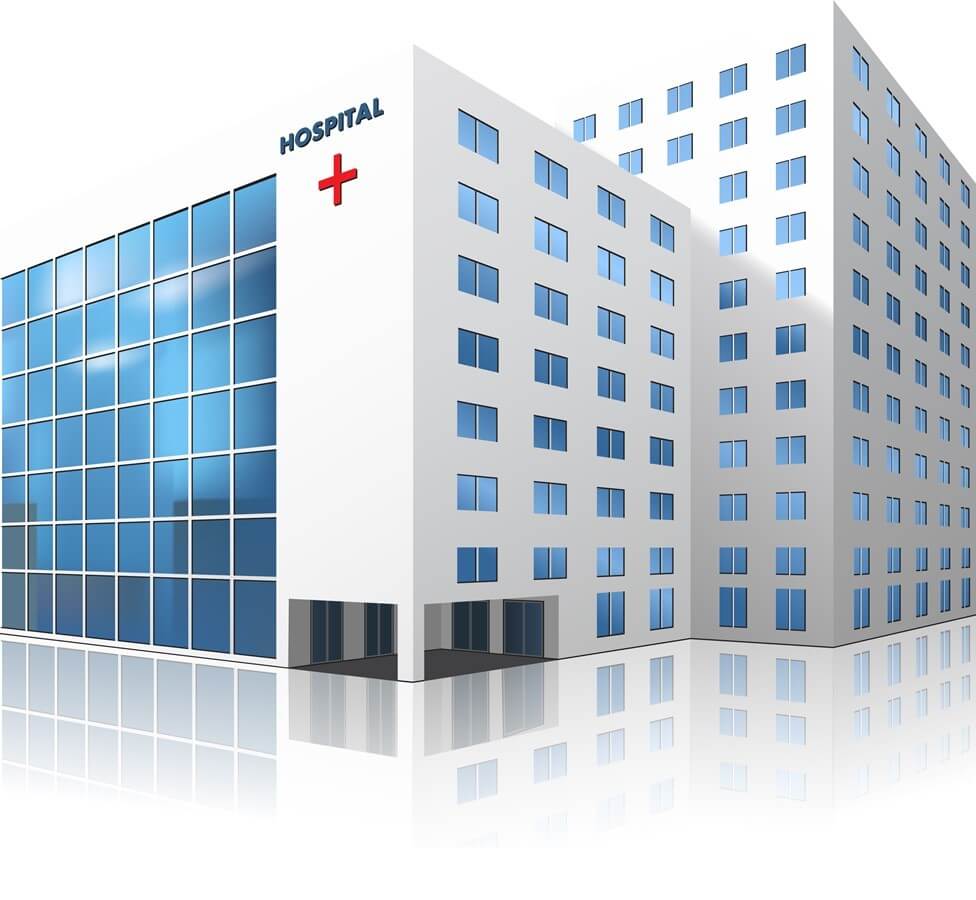
Studies are advancing on nisin's antimicrobial activities against MRSA, suggesting its potential as an alternative to traditional antibiotics. Similarly, nisin's effectiveness against VRE, another Gram-positive bacterium, has been recognized.
Nisin is particularly thought to be synergistic in removing biofilms formed on medical devices used in hospitals, a significant source of chronic infections. Biofilms in the food industry are known for harboring pathogens in hard-to-clean areas of production lines, leading to secondary contamination of food products.
For basic information on biofilms in the food industry, please refer to the article
Biofilm in food factories
In medical settings, biofilms on transplanted medical devices or damaged tissues cause persistent infections. Medical biofilms are a more critical issue than those in the food industry because bacteria within biofilms can resist antimicrobial concentrations over 100 times greater than those needed to kill non-biofilm bacteria. The efficacy of nisin against such biofilms has been reported and is under consideration for further use.
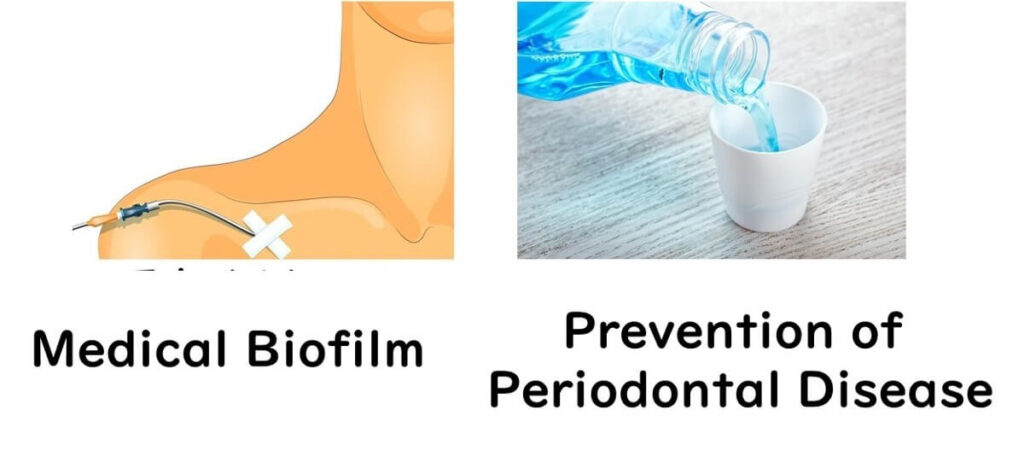
Furthermore, nisin is being explored for treating localized infections. Reports indicate its antimicrobial effects on conditions like mastitis, gastrointestinal infections, and skin infections. Nisin is also anticipated to prevent bacterial secondary infections in viral respiratory diseases. Additionally, its potential in dentistry, particularly in preventing periodontal disease, has led to increased interest, with developments in nisin-containing mouth rinses being particularly promising. There are also emerging reports of nisin's effectiveness in boosting human immune functions and specifically inhibiting the proliferation of cancer cells.
Thus, nisin's role extends far beyond its use as a food preservative, with wide-ranging applications in the medical field being increasingly explored. A search on the keyword "nisin" reveals a significant rise in the number of publications over the past decade, illustrating its growing importance and potential in healthcare.
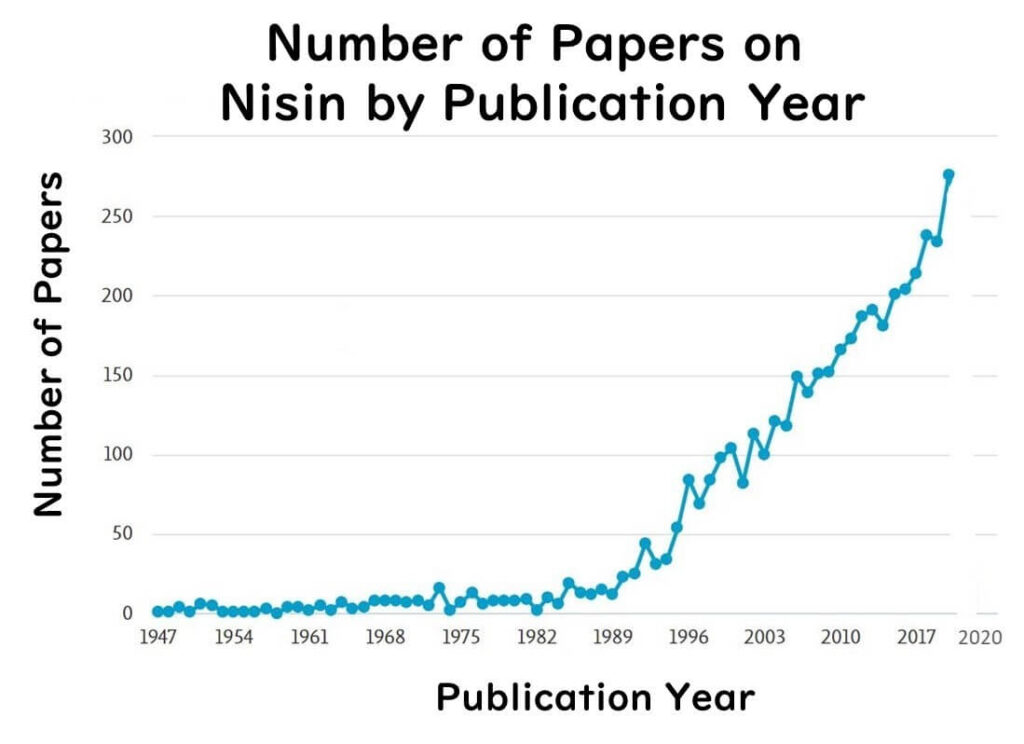
The Impact of "Preservative-Free" Perception on Nisin in Japan
Nisin was approved as a food additive in Japan in 2009, categorized under the mandatory "preservative" labeling. As discussed in the previous article, food additives required to display a "preservative" label often face consumer resistance in Japan.
For a deeper look into the current situation surrounding preservatives in Japan, refer to the below section
How Glycine Extends the Microbiological Shelf Life of Japanese Convenience Foods
Despite being widely used in Western countries, the adoption of nisin in Japan remains considerably limited even more than a decade after its approval.
In consultations I've had with food developers aiming to improve product shelf-life, the common premise is often to avoid using preservatives. For instance, this year, a food developer from a private company sought advice on controlling spoilage lactic acid bacteria in chilled foods. Controlling lactic acid bacteria is notoriously challenging, and internationally, nisin is considered the best solution. However, the developer preferred not to use additives labeled as preservatives due to domestic concerns.
For basic information on the spoilage issues with lactic acid bacteria, please see the article:
Lactic Acid Bacteria: More Than Just Beneficial Bugs in Foods
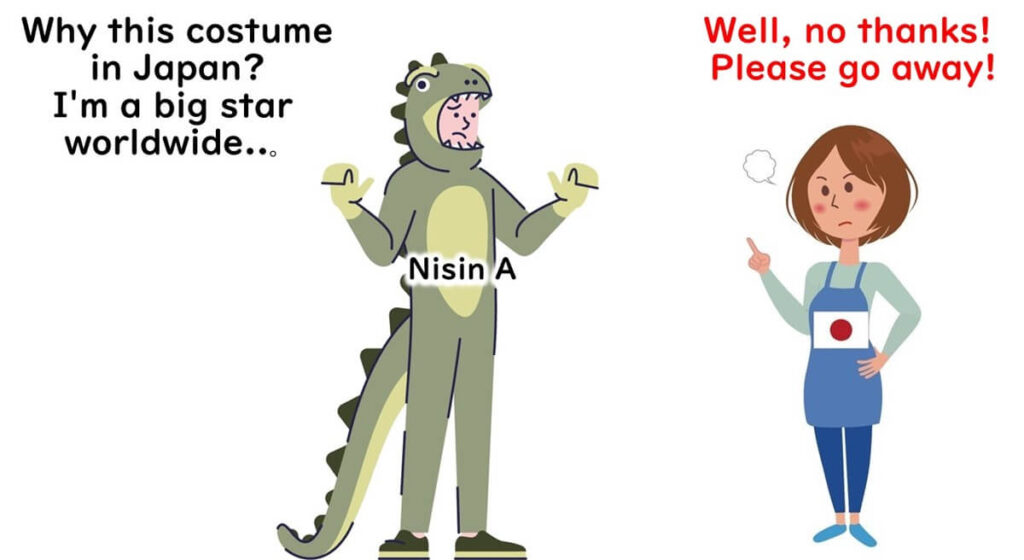
Such is the case in many discussions with private companies about microbial growth control; the examples are countless. This suggests a strong psychological reluctance among domestic food producers to use nisin because it must be labeled as a "preservative". The pervasive mindset in Japan seems to be "preservatives = disliked by consumers = better to avoid using them".
While writing this article, I watched a show on Netflix called "Famous in France", where a nationally beloved French comedian travels to the US West Coast to meet his teenage son from a previous marriage to an American woman. Despite his popularity in France, he is treated indifferently at Los Angeles airport, realizing his anonymity in the US.
Watching this film reminded me of nisin's situation. Like shirako and polylysine, which are primarily used in Japan and Asia, nisin, despite its global prevalence, is shunned in Japan due to its "preservative" categorization.
As I've mentioned in this and previous articles, nisin is an effective antimicrobial agent recognized globally as a natural preservative. It certainly deserves more acceptance in Japan, given its proven benefits and international standing.
Why Nisin is the Best Candidate for Controlling Spoilage Lactic Acid Bacteria
As noted earlier, there has been significant interest recently in controlling the proliferation of spoilage lactic acid bacteria. Among antimicrobial substances that can inhibit lactic acid bacteria, nisin, produced by closely related lactic acid bacteria, holds the highest potential. In other words, looking for alternatives might just be a roundabout way, making nisin the most straightforward option. Let's delve into a bit of biological explanation for this.
Here's a question I often pose to my students:
Question: If I suggested that you research Pseudomonas to combat Staphylococcus aureus when you entered the lab, what would you do?
Typically, students make a puzzled face, unsure whether to say Yes or No. I ask this question because I want them to first understand the natural role of bacteriocins as antimicrobials.
The answer to the above question is that such a research theme would be nonsensical. Why nonsensical? Because Pseudomonas has no necessity to produce antimicrobial substances against Staphylococcus aureus. Imagine a scenario in the human world where the winner of a 100-meter race wins 100 million yen, but second place and below get nothing. In the illustration below, a notably faster runner is confident of winning without bothering about the others.
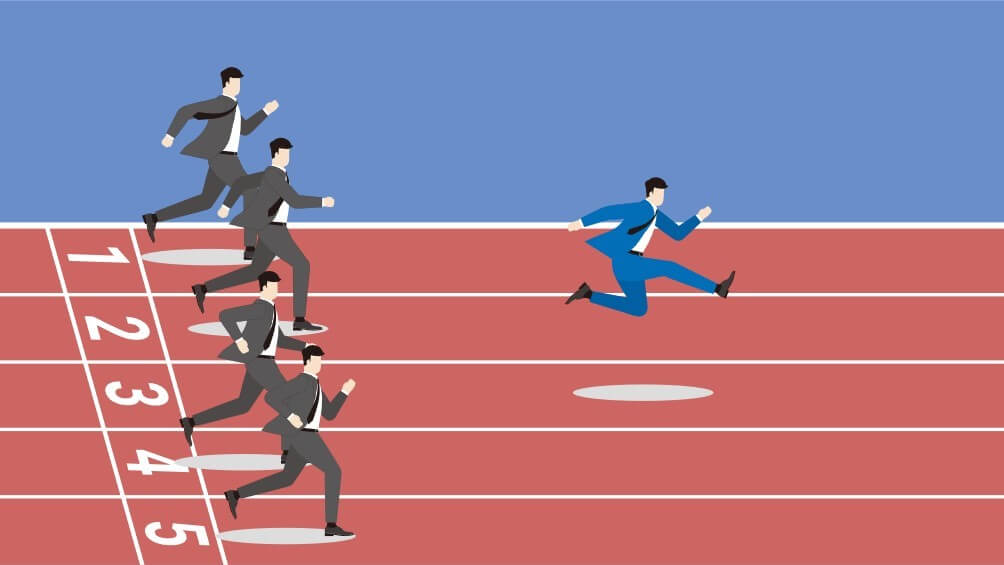
However, in another scenario, where a competitor might outrun the fastest runner, things could become dire. The leading runner, unsettled by the competition, might even resort to extreme measures like using a pistol to eliminate the second-place runner.
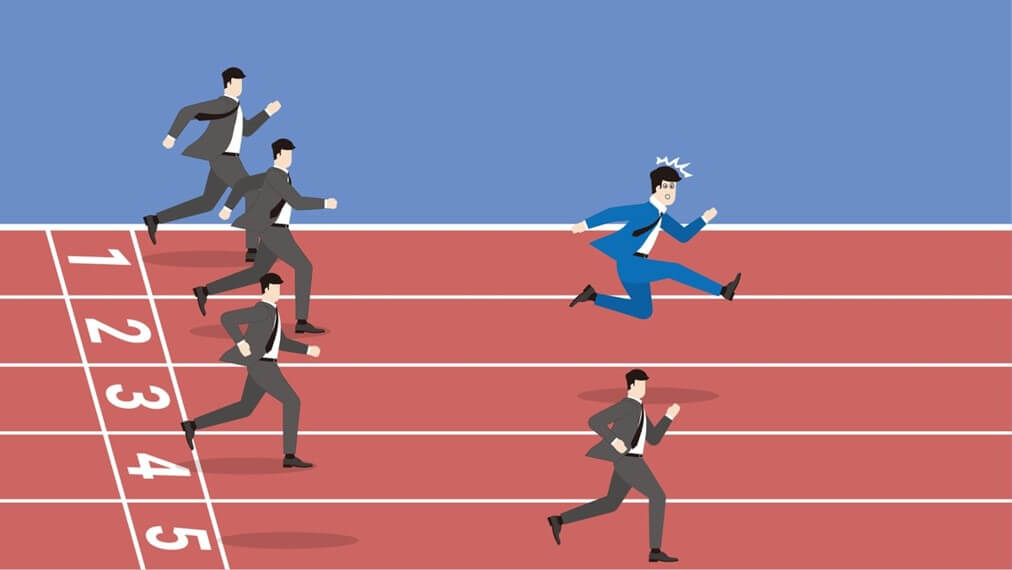
Translating this to the microbial world, a microbe that easily dominates its environment doesn't need to produce antimicrobials against other microbes. In our example, Pseudomonas thrives in oxygen-rich aqueous environments, while Staphylococcus aureus proliferates in salty, dry conditions. There's no need for Pseudomonas to target Staphylococcus aureus. If Pseudomonas were to produce an antimicrobial, it would likely target very similar microbes, essentially its direct competitors.
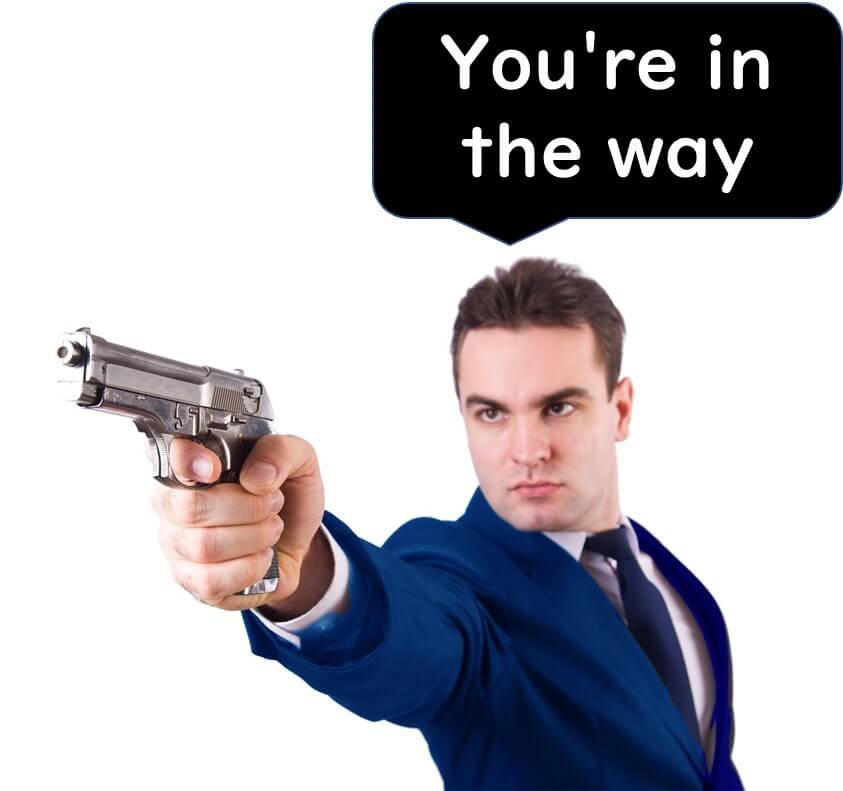
Hence, generally, when microbes produce antimicrobials, it is typical among closely related microbes, often used in a fight for survival in the same environmental niche. Nisin, as discussed in this article, is an antimicrobial substance used among lactic acid bacteria cohabiting the same environment to outcompete each other. Understanding the ecological aspects of such antimicrobial actions is crucial.